Course Directors:
Course Instructors:
Bill Kristan, Jr. (UCSD), Jimmy Liao (U. of Florida), Matthieu Louis (UCSB), Christopher Potter (Johns Hopkins), Pavan Ramdya (EPFL), Michael Reiser (HHMI-Janelia), Agnese Seminara (U. of Nice), Julie Simpson (UCSB), Marcus Stensmyr (Lund Univ.), Sebastian Streichan (UCSB), Tanya Tabachnik (ZMBBI, Columbia), Massimo Vergassola (UCSD), Daniel Wagenaar (Caltech), David Weisblat (UC Berkeley), and Claire Wyart (ICM Institute)
Guest Lecturers (partial list):
The summer course is closely linked to the concurrent KITP program Neural computations for sensory navigation: mechanisms, models, and biomimetic applications. The course participants will attend the program's daily research seminars as part of the course curriculum. Students and lecturers will also have frequent opportunities for less formal interactions. Confirmed participants include Jelle Atema (Boston U.), William Bialek (Princeton), Dimitry Chklovskii (Flatiron Inst.), Thierry Emonet (Yale), Vivek Jayaraman (HHMI-Janelia), Venkatesh Murthy (Harvard), Katherine Nagel (NYMC), Gonzalo Polavieja (Champalimaud), Sharad Ramanathan (Harvard), Dmitry Rinberg (NYM), Terrence Sejnowski (UCSD), Thomas Shimizu (AMOLF), Nathan Urban (U. of Pittsburgh), Jane Wang (Cornell), and Barbara Webb (Edinburgh U.). A complete list will be available in late March.
Course subject
Physics has become omnipresent in systems neuroscience: it plays a critical role in providing tools to record electrical activity of single neurons, monitor neural activity through multi-photon imaging, and model dynamical behavior of nonlinear systems of interconnected neurons. Neurophysics of Sensory Navigation will equip students with the conceptual and technical frameworks that physics and quantitative biology offer to study the integrated function of neural circuits. The school does not intend to provide a general introduction to systems neuroscience. It aims to be a creative research-oriented course closely linked to the KITP program Neural computations for sensory navigation: mechanisms, models, and biomimetic applications. In addition to their own intensive projects, participants will attend daily seminars of the KITP program, which will provide a broader perspective to the individual research projects conducted in the school.
The course targets graduate students and postdoctoral scholars with some research experience in systems and/or computational neuroscience. The course participants will form a diverse group with different academic backgrounds. We are especially eager to provide this opportunity to scientists from groups underrepresented in the sciences. Our goal is to permit experimentalists to familiarize themselves with theory and advanced data analysis while encouraging theorists to dive into wet-lab experimentation.
Experiments in systems neuroscience require a broad range of techniques, necessitating collaboration between scientists with complimentary areas of expertise. Accordingly, course participants will work in interdisciplinary teams that include both theorists and experimentalists, building common vocabulary and learning the physics and neurobiology that will help them tackle challenging systems neuroscience questions: how do noisy sensors reliably capture multisensory sensory information? How is the integration of sensory signals transformed into decision making? How are behaviors such as locomotion planned and controlled by the motor system? The course will center around research projects covering two themes: (1) mapping and functional characterization of neural circuits and (2) quantitative description and sensory manipulations of elementary behaviors. By working under the guidance of instructors and teaching assistants, students will be encouraged to develop and pursue their own questions.
Experimental Projects (partial list)
Bill Kristan, Jr.; Daniel Wagenaar; and David Weisblat: Tracking, modeling, and electrophysiological characterization of leech scanning behaviors
Most animals scan their environment to search for—among other things—food, potential mates, or places to hide. Leeches are excellent animals for studying scanning strategies because their behavior is robust and their nervous systems are accessible at the level of individual, identified neurons as they behave. Students will characterize scanning behaviors in two different leech species (one is carnivorous, the other sucks blood) to find common elements in leech behaviors as they crawl on substrates of different roughness and follow food clues such as chemical trails and water waves that generate both visual and tactile stimuli. From a detailed analysis of the scanning behaviors, the students will generate models based upon modular behavioral movements. They will then record electrically from identified neurons in the leech central nervous system to seek the neuronal mechanisms underlying different aspects of the scanning behavior. Ideally, these electrophysiological recordings will provide neuronal reality for their behavioral models, which can then be incorporated into biomimetic devices. Figure: time sequence micrographs and tracings of leech body position during a scanning behavior sequence
Pavan Ramdya and Julie Simpson: Creating virtual obstacles to study tactile navigation in adult flies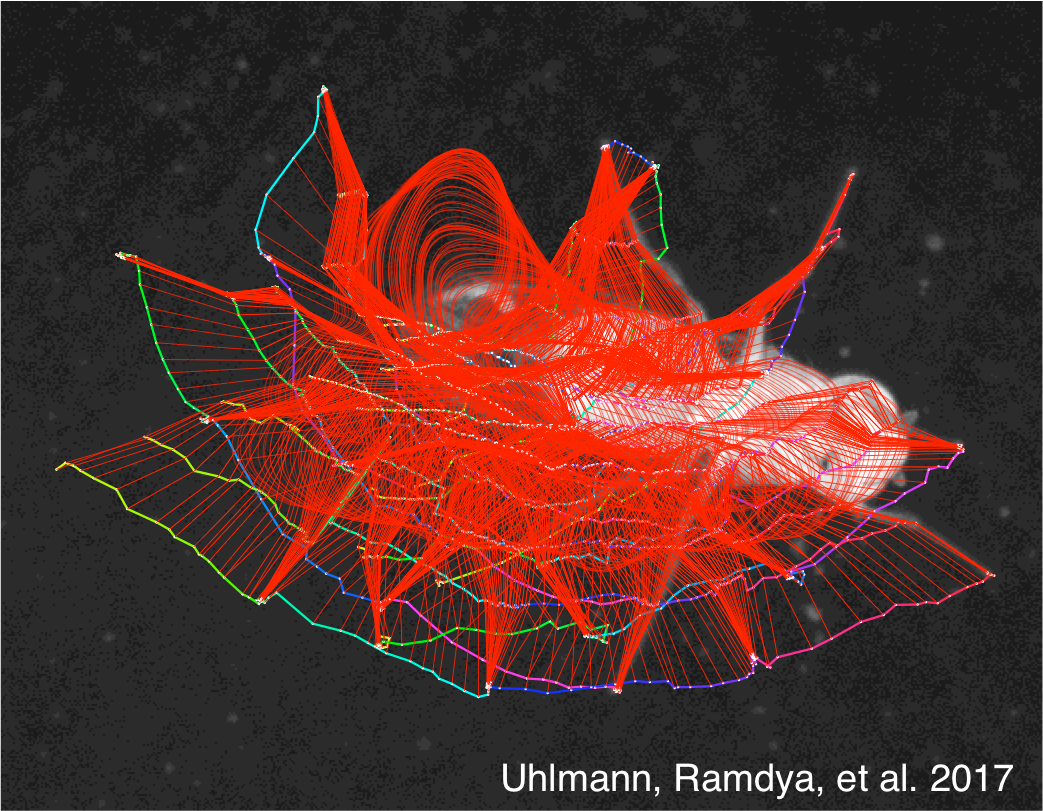
How do insects use mechanosensory information when detecting obstacles and exploring their environment? In this project, we will use limb-tracking software to analyze high-resolution behavioral videos of flies interacting with obstacles in real and optogenetically-generated virtual reality environments to identify the neural algorithms underlying mechanosensory navigation. Figure: Custom software is used to track the body and limb segments of fly while it is walking. This software will be used to quantify limb movements in response to real or virtual environmental obstacles.
Christopher Potter and Marcus Stensmyr: Modeling mosquito navigation behavior in response to humidity and temperature
Mosquitoes depend on temperature and humidity gradients to find suitable locations for egg-laying, to avoid inhospitable climates, and to find humans for a blood-meal. In this module, we investigate the behavioral interactions of adult and larval Aedes aegypti mosquitoes (vectors for Dengue, chikungunya, and Zika viruses) as they explore these crucial physical sensory stimuli. We will generate and test high resolution models linking temperature and moisture gradients with the behavioral movements of mosquitoes and use these to understand the strategies used by these insects to navigate these physical cues.
Matthieu Louis, Agnese Seminara, Sebastian Streichan, and Massimo Vergassola
The Drosophila larva has emerged as a powerful system to dissect the neural basis of sensory-driven behaviors. The larva demonstrates a rich repertoire of orientation responses under the control of a brain composed of fewer than 10,000 neurons that are being mapped in a wiring diagram. We will build an affordable (do-it-yourself) assay to track single larvae and to conduct closed-loop stimulation experiments with light. By optogenetically stimulating the larval olfactory system, we will create virtual olfactory realities. With this technology, we will apply a systems-identification approach to investigate principles underlying the sensorimotor control of odor-driven and light-driven behavior. Using light-sheet microscopy, we will also attempt to monitor the activity of the larval olfactory system during naturalistic food-search behavior. Figure: Remote-control of the trajectory of a Drosophila larva through the optogenetic manipulation of its olfactory system (head position in blue; centroid position in black).
Jimmy Liao and Claire Wyart: The kinematics of zebrafish orientation and navigation
Larvae and juvenile zebrafish exhibit robust orientation behaviors in response to chemical gradients and water flow. In this module, we will study the kinematics of locomotion of individual animals to understand how the initiation and direction of orientation responses is determined by the detection of olfactory cues and both laminar and vortical flows. Building on this analysis, we will examine how the presence of other conspecifics condition the orientation responses displayed by individuals.