Application deadline March 24: Rolling review begins March 17
Course Subject:
Mobile genetic elements (MEs) can mediate rapid adaptation by introducing genes that encode novel functions related to pathogenesis, symbiosis and metabolism into host cells. MEs, and the accessory genes they carry, are as varied as the hosts and niches they are found within. Fuller understanding of the dynamics, constraints and consequences of ME movement will require interdisciplinary research merging computational and experimental approaches. This hands-on course will integrate laboratory experiment with quantitative bioinformatic analysis, providing students from diverse disciplinary backgrounds with new experience and skills that will assist them in developing rigorous, quantitative research in microbial evolution and ecology.
Course Faculty:
Guest Lecturers (partial list):
The summer course is closely linked to the concurrent KITP program Horizontal Gene Transfer and Mobile Elements in Microbial Ecology and Evolution. Course participants will attend the program's daily research seminars as part of the course curriculum. Students and lecturers will also have frequent opportunities for less formal interactions. Confirmed program participants include Aude Bernheim (Pasteur Inst.), Devaki Bhaya (Carnegie Science/Stanford U.), Allison Carey (U. Utah), Ilana Brito (Cornell U.), Daniel Fisher (Stanford U.) Nandita Garud (Stanford U.), Graham Hatfull (U. Pittsburgh), Daniel Huson (U. Tübingen), Eugene Koonin (NCBI), Michael Laub (MIT), Katie Pollard (Gladstone Inst., UCSF), Martin Polz (U. Vienna), Paul Rainey (MPI-EB), Eduardo Rocha (Inst. Pasteur), Julia Salzman (Stanford U.), and Erik van Nimwegen (U. Basel).
Course Structure
The course is 5 weeks long, and runs concurrently with the KITP Program Horizontal Gene Transfer and Mobile Elements in Microbial Ecology and Evolution. Students will begin with a bootcamp on basic wetlab techniques, then break into project groups for the first section of wetlab projects. After wrapping up wetlab data collection and preparing samples for sequencing, students break for a drylab bootcamp. Students will then join and complete a drylab project. During this period, sequencing for wetlab projects will be completed. During the last week, students will analyse the data from their wetlab projects, and present their findings to the program.
The experimental projects are led by instructors and TAs working closely with groups of 4-5 students. Additional guest lectures and training modules will be provided throughout the course. Students regularly attend morning lectures offered as part of the KITP Program, then head off for lab work in the afternoon and evening. Students are welcome to join visiting lecturers and program participants for tea breaks and evening BBQs at the Munger Physics Residence. Weekends are open for students to explore and enjoy the many recreational opportunities in the Santa Barbara area.
Accommodations, Fees, and Financial Assistance
There is no tuition, lab fee, or room and board fee. Admitted students are provided with double-occupancy rooms in UCSB student apartments and a 19-meal plan at campus dining commons. A limited number of single rooms are available for a $2064 supplement. Students who need financial assistance with travel expenses can request it in the application form; financial need does not affect an applicant's chances of admission.
Experimental Projects
Evolution of bacterial genomic structure (Richard Neher and Marco Molari)
Bacteria exchange genetic material horizontally, duplicate DNA, delete parts or invert stretches of their genome resulting in complex patterns of genetic diversity. Despite the overall diversity and structural complexity, the order of essential genes in bacterial genomes tends to be highly conserved and most of the horizontally acquired diversity is concentrated in hot-spots. In this project, we will construct genome graphs of groups of recently diverged bacteria (specific sequence types) using PanGraph. PanGraph is a tool to detect shared homologous sequences between a large number of genomes and summarize it in a concise graph structure. Using this graph, we will quantify the rates at which horizontal processes add or exchange genes and will detect aforementioned hot-spots and characterize their sequence repertoire.
___________________________________________________________________________________________________________
Examining G x G x E interactions in evolution of bacterial-pathogen resistance to therapeutic phages (Paul Turner)
This project examines three lytic phages of Pseudomonas aeruginosa (PsA) that have been used successfully in FDA-approved emergency therapy to treat antibiotic-resistant pulmonary infections in humans. Each phage infects PsA using a different cellular receptor, and can select for host bacteria to evolve phage-resistance that either reduces virulence or switches hosts to become antibiotic sensitive (i.e., phage induced trade-offs). In week 1, students will challenge the phages to infect a collection of PsA strains that are cultured in different environments, to test how the culture conditions impact the spectrum of isolated phage-resistant mutants. These ‘rescue’ mutants will then be submitted for whole-genome sequencing, to analyze the loci underlying evolved phage-resistance. Week 2 will involve analyses of the genomic results. In addition, students will conduct phenotypic (growth) assays on the mutant isolates using automated spectrophotometers (microplate readers) and R programs that infer quantifiable bacterial traits, to conduct rigorous genotype-phenotype analyses. The overall goal is to generate a large dataset of novel results that will inform phage-bacteria-environment (G x G x E) interactions, useful for future emergency patient treatment leveraging phage-induced trade-offs in this important bacterial pathogen system.
___________________________________________________________________________________________________________
Dynamics of ICE movement in bacterial populations (Elena Colombi and Honour Mc Cann)
Integrative and conjugative elements (ICEs) are large, self-transmissible mobile elements that mediate the movement of ecologically significant genes across even distantly related membes of the Pseudomonas syringae species complex. Excision, conjugal transfer from the donor, and site-specific integration into the recipient can occur within fifteen minutes in vitro. Newly acquired ICEs can replace or recombine with ICEs already present in recipient cells, generating novel chimeric elements. ICEs are known to vector genes with functions associated with antibiotic resistance, pathogenicity and symbiosis, bacteriocin synthesis and more. For this project, we will start by performing transfer and competition experiments to assess the host range and fitness contributions of ICEs in different recipient strains. We will then set up and sequence a serial passage experiment to quantify rates of ICE transfer in mixed populations. Students will explore whether ICE proliferation is linked to their contributions to host fitness, or is selfish in nature.
___________________________________________________________________________________________________________
Rates of Horizontal Gene Transfer and Transposition in Non-Growing Bacteria (Roberto Kolter and Benedek Facskó)
Comparative genomics led to the insight that horizontal gene transfer events are the prevalent mode of bacterial genome evolution. We know much about the modes of horizontal gene transfer, i.e., transformation, conjugation, transduction, etc. Most of the past studies that measured the rates of these processes utilized fast growing populations. Yet, we know that in natural settings, bacteria seldom encounter conditions propitious for fast growth. In this project, we will quantitate the rates of transduction in non-growing or very slow-growing bacterial populations. As a complement, we will also follow the dynamics of mobile genetic elements in non-growing cells. In addition, we'll explore the ecological implications of the little-explored phenomenon of abortive transduction.
___________________________________________________________________________________________________________
Spatial patterns of soil viral communities (Joanne Emerson)
Students will investigate spatial structuring of soil viral communities to identify patterns and drivers of community composition. The project will begin with sampling soil and wetland environments in the area, then enriching for viral communities via filtration. Students will extract DNA from filtered and unfiltered samples, then sequence the viromes and metagenomes using MinION and Illumina platforms. Viral genomes will then be identified, and viral community composition will be compared to identify ecological differences along the sampled gradients. Finally, students will look for evidence of horizontal transfer by searching for integrated prophages in microbial genomes and 'host' genes in viromes.
Application deadline: February 26, 2023
Course Subject:
Animal morphogenesis, the question of how body parts acquire their form, remains a fascinating open problem of developmental biology and physics. Molecular analysis uncovered how the basic architecture of the body plan is laid out and how genetic patterning instructs cell fate. Quantitative investigation of cellular flow in vivo indicates that feedback mechanisms between genetics, mechanical force generation, and geometry ensure a robust and reproducible time course of shape. These analyses rely on studying embryogenesis in model organisms such as the fruit fly, mouse, or zebrafish. While superb genetic perturbations are readily available, physical analysis, such as measurements or even perturbation of mechanics, remains a high hurdle.
Cell biology successfully incorporated experimental methods from physics and engineering into the study of immortalized cell lines. Culturing techniques enable precise regulation and measurements of biochemical factors, as well as physical, and geometric constraints. Recent progress with stem cell culture now enables addressing fundamental questions of developmental biology, about the interplay of mechanics, biochemical patterning, and geometry. This course will study synthetic morphogenesis by integrating quantitative data analysis and mathematical modeling, in a variety of hands-on projects. Experimental systems include the mechanobiology of human embryonic stem cell patterning, mechanical pattern formation in the avian dermis, synthetic morphogenesis with mouse as well as human embryonic stem cell lines, and the regenerative model system hydra.
Course Directors:
Instructors:
Idse Heemskerk (Univ. of Michigan), Kinneret Keren (Technion), Alan Rodrigues (Rockefeller ), Amy Shyer (Rockefeller), and Vikas Trivedi (EMBL Barcelona)
Guest Lecturers (preliminary list):
The summer course is closely linked to the concurrent KITP program Dynamics of Self-Organization in Animal and Plant Development. Course participants will attend the program's daily research seminars as part of the course curriculum. Students and lecturers will also have frequent opportunities for less formal interactions. Confirmed program participants include Dominique Bergmann (Stanford), James Briscoe (Crick Inst.), Francis Corson (ENS Paris), Cassandra Extavour (Harvard), Ken Irvine (Rutgers), Thomas Lecuit (College de France/IBDM), Ani Kicheva (IST Austria), Prisca Liberali (FMI), Adam Martin (MIT), Elliot Meyerowitz (Caltech), Rashmi Priya (Crick Inst.), Sharad Ramanathan (Harvard), Jochen Rink (MPI-NAT), Adrienne Roeder (Cornell), Timothy Saunders (Warwick), Eric Siggia (Rockefeller), Katharina Sonnen (Hubrecht Inst.), David Sprinzak (Tel Aviv), and Aryeh Warmflash (Rice).
Course Structure
Students will attend Dynamics of Self-Organization in Animal and Plant Development program talks each morning and spend afternoons and evenings working in their lab groups and attending modeling and analysis tutorials. Interaction with program speakers will also be facilitated through tutorial sessions and informal meetings over coffee and meals, as well as social evenings held jointly with the program. Students will be matched to experimental modules based on their ranked choices and have ample room to explore their own interests, based on initial instructor-guided projects. Students in different modules will interact informally – many research areas benefit from comparison or use similar technical approaches. Students teach each other, sharing their different expertise--project groups will intentionally include students from different academic backgrounds--and work closely with expert instructors, TAs. and guest lecturers from the associated KITP program.
There is no course fee, and students are provided with housing at UCSB's Manzanita Village residence halls and a 19-meal plan at campus dining commons. A typical day's schedule includes:
- 2 morning talks at KITP
- Lunch in a dining hall or campus cafe, with other course or program participants
- Afternoon lab work
- Dinner in a dining hall, followed by evening lab work and/or science discussions
On weekends, students can attend program BBQs and self-organize outings to the beach or around Santa Barbara.
Experimental Projects (partial list)
Amy Shyer and Alan Rodrigues: Morphological symmetry breaking in self-organizing mesenchymal tissue
While much of the recent advances in our understanding of mechanics in development have focused on epithelial tissues, the Shyer-Rodrigues Laboratory of Morphogenesis studies morphological symmetry breaking driven by self-organizing mesenchymal tissues. Using a first-of-its-kind experimental platform, we combine live-cell imaging with collagen substrates specifically engineered to enable skin progenitor cells to self-organize into follicle patterns (https://www.quantamagazine.org/embryo-cells-set-patterns-for-growth-by-pushing-and-pulling-20220712/). By recapitulating a dynamic in vivo process, we will observe and manipulate multicellular activity in a tissue as it is generated.
___________________________________________________________________________________________________________
Idse Heemskerk: Predicting cell fate in pluripotent stem cells
Figure: left: cells stained for fate markers after 42h of differentiation, right: (partially hypothetical) probability distributions showing how different factors relate to fate
Pluripotent stem cells have remarkable capacity to self-organize into heterogeneous structures consisting of multiple cell types. Quantitative understanding of what makes one cell differentiate to one fate and a neighboring cell to a different fate is in most cases lacking. Variations in initial cell state, differences in morphogen signaling, and the mechanical environment all contribute, but their relative contributions and interdependence are often difficult to dissect.
In the context of the first cell fate decisions after pluripotency loss, associated with gastrulation, we will determine using single cell analysis of live and fixed imaging data how much redundant and non-redundant information different factors contain about cell fate. For example: does cell geometry at the onset of differentiation predict fate? What about the morphogen signaling dynamics? If so, does geometry predict morphogen signaling dynamics or are they independent inputs? And how do these relationships depend on substrate stiffness and intercellular forces?
Left figure: cells stained for fate markers after 42h of differentiation. Right figures: (partially hypothetical) probability distributions showing how different factors relate to fate. Figures by Seth Teague, unpublished.
___________________________________________________________________________________________________________
Kinneret Keren: Cytoskeletal dynamics in regenerating Hydra
Figure: The supracellular actin fibers in a small regenerated Hydra (green) plotted together with the orientation field (black lines) and the nematic order parameter (jet colors).
Hydra is a small fresh-water animal renowned for its extraordinary whole-body regeneration capabilities. We will study the emergence of large-scale order in the cytoskeleton of regenerating Hydra, focusing on the establishment of parallel arrays of supracellular contractile actomyosin fibers. We will excise tissue pieces from transgenic Hydra expressing lifeact-GFP in the ectoderm and/or endoderm, and follow the dynamic organization of the actin cytoskeleton during regeneration using live microscopy. We are particularly interested in understanding how the large-scale nematic order develops in orthogonal directions in the two epithelial tissue layers, and how this relates to the morphogenesis process.
___________________________________________________________________________________________________________
Vikas Trivedi: Biophysics and metabolism in self-organising structures from embryonic stem cells
Figure: Hybridisation chain reaction (HCR) staining of 96h gastruloids. RNA expression patterns of Brachyury (green - primitive streak/mesodermal), Sox2 (red - pluripotent/ectodermal) and Sox17 (yellow - endodermal). The white line indicates the anterior-posterior axis illustrating the spatial organisation of cell fates in the gastruloid model.
How can tissue shapes and patterns emerge reproducibly and robustly in multicellular systems like animals? Despite more than 100 years of embryology, it still remains unclear how gene networks, forces and mechanical properties integrate with the metabolic state of the cells to self-organize complex structures. This is due to our inability to disentangle the combined action of these factors (biophysical properties, gene networks and metabolic activity) within populations of genetically equivalent cells. For example, the activity of genetic programs is accompanied by emergence of distinct biophysical characteristics within different cell types. At the same time the metabolic state of a cell can influence cell fate decisions by modifying transcription factor signalling, the epigenetic landscape as well as cellular mechanics. Making quantitative measurements and systematic perturbations of these factors are nearly impossible in vivo. Therefore, we will take advantage of aggregates of mouse embryonic stem cells that recapitulate hallmarks of early embryonic development in vitro. This will allow us to explore the role of metabolism in symmetry breaking, germ layer specification and emergence of distinct biophysical properties that mould the tissue. We will use machine-learning based image analysis, quantitative measurements of relative material properties and genetically engineered cells with live imaging to dissect how coordinated changes in metabolic states and biophysical properties affect morphogenesis.
___________________________________________________________________________________________________________
Idse Heemskerk, Sebastian Streichan and Xavi Trepat: Linking fate and force in micro patterned human pluripotent stem cells
Micropatterned colonies of human pluripotent stem cells (hPSCs) recapitulate aspects of human embryonic development. Such systems provide an opportunity to study developmental events that are otherwise inaccessible to quantitative experimental approaches. This cell culture environment offers new interrogation and perturbation approaches that promise insight into the dynamics early human morphogenesis. These include spatial force measurement maps, tuning substrate stiffness, and shape as well as size of the micropatterned hPSCs colonies. We seek to exploit these advantages and leverage synergies between groups to study the interplay of biochemical signaling, tissue mechanics, and geometry. Specifically, we will have a project studying how the interplay between secreted factors and mechanical forces drives the formation of primordial germ cells in humans. Another example will investigate how mechanical stresses are coordinated to achieve neural plate folding into a tube.
Applications are being accepted and reviewed on a rolling basis until April 15.
In order to allow time for visa processing, international scholars in particular should submit their applications as early as possible.
Course Subject:
Animals interact with the world through movement. Crawling, swimming, walking, and flying animals face different challenges. Using a range of experimental techniques, we will explore locomotor behavior, considering contributions from the nervous system and musculature, and confronting biomechanical constraints for creatures with 0 to 8 limbs in environmental conditions ranging from sea to sky.
Experiments will be organized in team-taught modules based on comparative analysis of between tractable model organisms with different means of locomotion.
Course Directors:
Instructors:
Serena Ding (MPI, Konstanz), Pavan Ramdya (EPFL), Jane Loveless (OIST), Jimmy Liao (Whitney Lab, U. Florida), Jessica Fox (Case Western Reserve), Eugenia Chiappe (CNP), Ellie Heckscher (U. Chicago), Tanya Tabachnik (Zuckerman Inst., Columbia), Andrew Straw (U. Freiburg), and Brad Dickerson (Princeton)
Guest Lecturers (preliminary list):
The summer course is closely linked to the concurrent KITP program Neurophysics of Locomotion. Course participants will attend the program's daily research seminars as part of the course curriculum. Students and lecturers will also have frequent opportunities for less formal interactions. Confirmed program participants include Jimena Berni (U. Sussex), Barry Dickson (U. Queensland), Akinao Nose (U. Tokyo), Dan Tracey (U. Indiana), Dmitri Chklovskii (Flatiron Inst. & NYU), Andrew Leifer (Princeton), David Schoppik (NYU), John Tuthill (U. Washington), Michael Dickinson (Caltech), Kathy Nagel (NYU), Simon Sponberg (Georgia Tech), Madhu Venkadesan (Yale), Ilya Nemenman (Emory), Greg Stephens (VU Amsterdam & OIST).
Course Structure
The structure for the five-week school follows the model established in the 2018 course on sensory navigation. The course will be closely linked to the concurrent Neurophysics of Locomotion program, with students attending program talks each morning, as well as joint social events. Interaction with program speakers will also be facilitated through tutorial sessions and informal meetings over coffee and meals. Students will be matched to experimental modules based on their ranked choices and have ample room to explore their own interests, based on initial instructor-guided projects. Students in different modules will interact informally – many research areas benefit from comparison or use similar technical approaches. Students teach each other, sharing their different expertise--project groups will intentionally include students from different academic backgrounds--and work closely with expert instructors, TAs. and guest lecturers from the associated KITP program.
There is no course fee, and students are provided with housing at UCSB's Santa Ynez apartments and a 14-meal plan at UCSB dining halls. A typical day's schedule includes:
- 2 morning talks at KITP
- Group lunch in a dining hall, or pizza at KITP with informal science discussion or tutorials on topics of interest
- Afternoon lab work
- Dinner in a dining hall, followed by evening lab work and science discussions
On weekends, students can attend program BBQs and self-organize outings to the beach or around Santa Barbara.
Experimental Projects (partial list)
Monika Scholz and Serena Ding: Caenorhabditis elegans locomotion in environments with different physical properties
Locomotion is intimately connected to the substrate on or in which it occurs. A swimmer in a liquid environment with drag needs to perform different strokes to effectively move its body than a walker on a hard surface. Using the roundworm C. elegans as a model, we will explore the interaction between the nervous system, muscular contractions and different substrates of locomotion in 2D and 3D motion. By altering the physical environment that the animals move in, we will explore how locomotion patterns and the underlying neural signals are altered by the environment using behavioral tracking and calcium imaging.
Figure: Tracks of C. elegans on soft (2% agarose) and stiff (10% agarose) substrates.
___________________________________________________________________________________________________________
Jessica Fox and Brad Dickerson: Sensory integration for fly flight
Locomotion requires animals to integrate external signals from the environment with internal signals about their own head and body movements. This is a challenge for walking animals, and becomes even more complex during high-speed, three-dimensional flight. We will use quantitative behavioral analysis, optogenetic manipulations, and neural imaging to study how fruit flies (Drosophila melanogaster) integrate visual and mechanosensory signals to control their flight. Because both vision and mechanosensation are active senses during fly flight, we will explore neuromuscular control in both the head (for gaze control) and the haltere (for mechanosensory feedback).
Figure: A) Location and structure of the Drosophila haltere. B) Schematic of the control loop linking visual and mechanosensory feedback for fly flight.
___________________________________________________________________________________________________________
Ellie Heckscher, Matthieu Louis, and Jane Loveless: Evolution of the neuromechanics of locomotion in the Drosophila larva
At the larval stage, Drosophila move through waves of peristaltic contractions that travel from the posterior (tail) to the anterior (head) end of the body. The execution of these waves is rhythmically organized across body segments. While symmetrical contractions produce forward or backward locomotion, asymmetric contractions underlie turning maneuvers and active sampling through sweeping movements of the head. How peristalsis emerges from the interplay between the biomechanics of the larval musculature and its nervous system remains elusive. We will approach this question by comparing the locomotor behaviors and neuromusculatures of different species of the Drosophila group (and beyond). In particular, we will examine whether and how evolution has modified the control of peristaltic locomotion to adapt to the environmental properties of distinct ecological niches.
Figure: Drosophila larva (left) and examples of larval trajectories of four Drosophila species on an agarose substrate with the same stiffness (Elie Fink, Louis lab).
___________________________________________________________________________________________________________
Jimmy Liao and Claire Wyart: Long-scale patterns in the kinematics of fish during navigation, hydrodynamics of schooling, and optomotor responses
Larvae and juvenile zebrafish exhibit robust orientation behaviors in response to visual gradients and water flow. In this module, we will investigate the long-scale kinematics of locomotion of fish navigating in visual or flow gradients to understand how patterns emerge in either single individuals or schools of fish. We will compare the effects of the presence of conspecifics to visual and acoustovestibular stimuli eliciting escape responses in single individuals. Building on this analysis, we will examine how the presence of other conspecifics influence the long-scale dynamics displayed by individuals.
___________________________________________________________________________________________________________
Eugenia Chiappe, Pavan Ramdya, Julie Simpson, and Andrew Straw: How Flies Walk: exploring behavioral algorithms for sensory control of legged locomotion over complex terrain using adult Drosophila
Flies coordinate six legs to walk, cross gaps, and climb over obstacles. They display a range of gaits and exploratory movements but the full behavioral repertoire and underlying neural circuits are not fully understood. Visual, mechanosensory, and proprioceptive inputs contribute to the limb coordination required. We will assess flies’ capacity for motor learning using an obstacle course or treadmill to measure spatial memory and locomotor strategies under different sensory conditions. This module will use quantitative behavioral analyses and optogenetic perturbations to probe the mechanisms of walking in flies, in particular how sensory feedback and central pattern generators may underlie locomotor control.
Walking fly figures: Ramdya lab (top) and Chiappe lab (bottom)
Application deadline: February 15, 2021.
Course Directors:
Instructors:
Martina dal Bello (MIT), Jonathan Friedman (Hebrew U), Josh Goldford (MIT), Ben Good (Stanford), Akshit Goyal (MIT), Tami Liberman (MIT), Pankaj Mehta (BU), Julian Regalado Perez (U. Copenhagen), Joshua Weitz (Georgia Tech)
Guest Lecturers (preliminary list):
The summer course is closely linked to the concurrent KITP program The Ecology and Evolution of Microbial Communities. Course participants will attend the program's daily research seminars as part of the course curriculum. Students and lecturers will also have frequent opportunities for less formal interactions. Confirmed program participants include Devaki Bhaya (Carnegie Inst.), Daniel Fisher (Stanford), Susan Holmes (Stanford), Terry Hwa (UCSD), Jay Lennon (Indiana U), Sergey Maslov (UIUC), Pankaj Mehta (Boston U.), Andrew Murray (Harvard), Diane Newman (Caltech), Alex Petroff (Clark U), Paul Rainey (Max Planck), Alvaro Sanchez (Yale), Daniel Segrè (Boston U.), Wenying Shou (UCL), Kim Sneppen (Bohr Inst.), Alfred Spormann (Stanford), Ned Wingreen (Princeton)
Course Subject
Microbes form diverse and complex communities in both environmental and host-associated niches. These communities are ubiquitous and have global impacts on processes as diverse as biogeochemical nutrient cycling in terrestrial and marine ecosystems; the formation of intimate associations with varied hosts, and the suppression or emergence of disease. This hands-on research course will integrate laboratory projects and mathematical modelling to provide students with an understanding of the new tools and analytical approaches used to address questions in microbiome ecology and evolution. Students will gain experience developing models and reproducible analysis workflows to ask sharp, quantitative research questions focused on the discovery of the principles underlying microbial community structure, function and evolution.
Online Course Structure
Because of campus and lab access restrictions we have redesigned the course converting it to a “dry-lab” form. The course will run in remote mode via Zoom. Our course will be based on “paper projects” that will involve in-depth study (including data re-analysis) of published work under the guidance of its authors. The first week will focus on tutorials building up basic skills required/advantageous for one or more “paper projects”. Weeks 2 and 3 are allocated for two-week long paper projects. Three projects will run in parallel with students assigned to different groups for their duration. Weeks 4 and 5 will offer a different set of one-week projects/active journal clubs. The basic expectation is for the projects to include six (or more) hours of Zoom time “in class" per week, for example on Monday, Wednesday and Friday. The students will be expected to listen to the concurrently running Eco/Evo program lectures, Zoom among themselves or work independently the rest of the time.
"Dry-lab" Projects (partial list)
Two-week projects:
Ben Good (Week 2-3): G Garud, B. H. Good, O. Hallatschek, and K. S. Pollard. Evolutionary dynamics of bacteria in the gut microbiome within and across hosts. PLoS Biology 17(1):e3000102 (2019).
This module will introduce approaches for tracking genetic variation within species in complex metagenomic samples. We will explore these questions in the context of the human gut microbiome, by reproducing key results from our recent study (Garud, Good et al, PLoS Bio 2019). We will start from the raw sequencing reads, and slowly build up approaches for detecting within-host evolution and strain replacement events in longitudinally sequenced fecal samples. Our goal will be to understand some of the main challenges involved in working with metagenomic datasets, and how they both constrain and enhance the evolutionary inferences that can be drawn from them.
Key references:
1. Garud & Good et al (2019). Evolutionary dynamics of bacteria in the gut microbiome within and across hosts. PLoS Biology 17(1):e3000102.
https://journals.plos.org/plosbiology/article?id=10.1371/journal.pbio.3000102
2. Roodgar & Good et al (biorXiv). Longitudinal linked read sequencing reveals ecological and evolutionary responses of a human gut microbiome during antibiotic treatment. https://www.biorxiv.org/content/10.1101/2019.12.21.886093v2
Background reading:
We will try to introduce all of the necessary concepts as we go, but students looking for additional background may find the following resources helpful.
(1) A. Murat Eren, Microbial ‘Omics: An Introduction. https://merenlab.org/momics/
(2) Nayfach et al (2016). An integrated metagenomics pipeline for strain profiling reveals novel patterns of bacterial transmission and biogeography. Genome Res, 26(11):1612-1625.
https://pubmed.ncbi.nlm.nih.gov/27803195/
(3) B.H. Good. Lecture Notes on Quantitative Evolutionary Dynamics and Genomics. https://bgoodlab.github.io/courses/apphys23x7/.
___________________________________________________________________________________________________________
Jonathan Friedman & P. Blainey (Week 2-3): Positive interactions are common among culturable bacteria. bioRxiv 2020.06.24.169474
https://doi.org/10.1101/2020.06.24.169474.
This module will be on (pairwise) interactions between bacteria. I will discuss how to infer interactions from species abundance data, and the participants will analyze data to study how interactions change across environmental conditions, and as species coevolve. The specifics necessarily depend on the participants' background, what was covered in the first-week tutorials, and the amount of time they have to dedicate to working on this. This is a nice introductory paper to inferring interactions, and the data we will analyze is from our preprint using the kChip to study interactions across carbon sources, and paper about species coevolution.
___________________________________________________________________________________________________________
Seppe Kuehn (Week 2-3): Predicting metabolite dynamics from genomic structure in denitrifying bacterial communities.
Project will follow and extend our recent preprint https://www.biorxiv.org/content/10.1101/2020.09.29.315713v2
The goal of this project is to address the ‘structure-function’ problem in communities. How does the genomic structure determine metabolic function? We pose this question as a prediction problem: can we predict the dynamic flux of metabolites through a consortium from the genomes of each member of the community? We use denitrification as a model process - which is anaerobic respiration utilizing oxidized nitrogen compounds as electron acceptors. We will infer consumer resource model parameters from time series measurements of metabolites (data provided). We will use these models to predict metabolite dynamics in consortia of multiple strains and then compare those predictions with data. Finally, we will take a statistical approach using regularized regression to map the genes each strain possesses to their associated consumer resource model parameters - completing a map from genomes to community-level metabolic function. Tutorials on consumer resource models and python will be sufficient to participate in this project. We will direct students towards open questions which emerge from the study above and provide raw data
One-week projects:
Joshua Weitz (Week 4): Viral Strategies and Dynamics from Lysis to Latency
Objective: Viral infections can lead to the lysis of microbes and the release of organic matter back into the environment. This organic matter can then be taken up by other organisms, promoting new growth (e.g., by heterotrophic bacteria). Yet, not all viral infections end in lysis. Temperate bacteriophage can integrate their genomes into the chromosome of the bacterial host forming a lysogen. The integrated phage genome (or ‘prophage’) can be stably passed from mother to daughter cells. Over time, the prophage can also induce, reinitiating the lytic pathway, leading to the lysis of the bacterial host and the release of virions back into the environment. Notably, both the ‘decision’ to lyse or integrate upon infection and the ‘decision’ to induce from the lysogenic state are modulated by viral, cellular, and environmental factors. The aim of this short course is to develop a foundational theoretical framework as well as practical computational skills to analyze such decisions in a dynamical systems context. To do so, the short course will provide students with the necessary ecological, theoretical, and computational foundations to explore problems independently. The course will include background information on nonlinear dynamical systems, local stability analysis, invasion analysis, as well as core computational methods for analyzing the joint dynamics of viruses and their microbial hosts. Students are expected to work independently or in small groups to analyze how the invasion fitness of viral strategies depends on ecological context and how distinct viral strategies interact.
________________________________________________________________________________
Tami Lieberman and Shijie Zhao (Week 5): Adaptive Evolution within Gut Microbiomes.
https://www.sciencedirect.com/science/article/pii/S1931312819301593
More details to follow
________________________________________________________________________________
Martina Dal Bello et al. (Week 4) Resource-diversity relationships in bacterial communities reflect the network structure of microbial metabolism https://www.biorxiv.org/content/10.1101/2020.09.12.294660v2
More details to follow
________________________________________________________________________________
Akshit Goyal, Otto Cordero & Sergey Maslov (Week 5?)- Predicting community metabolites and interactions using models and data
Based on: Goyal et. al., Ecology-guided prediction of cross-feeding interactions in the human gut microbiome, Nature Communications (2021). https://www.nature.com/articles/s41467-021-21586-6
More details to follow
________________________________________________________________________________
Goldford et al. Emergent simplicity in microbial community assembly. Science. 2018 Aug 3;361(6401):469-474.
https://www.ncbi.nlm.nih.gov/pmc/articles/PMC6405290/
More details to follow
________________________________________________________________________________________
Additional resources for students:
https://merenlab.org/2020/06/27/seminar-series-on-microbial-omics/
https://online.umich.edu/series/python-for-everybody/
https://www.amazon.com/Computing-Skills-Biologists-Stefano-Allesina/dp/0691182752
Lectures on Bacteriophages: KITP Bacteriophage forum
Lectures on Microbial Metabolism: KITP forum on Microbial Metabolism
________________________________________________________________________________________
Application: deadline March 10, 2019
Letters of recommendation: two per applicant, deadline March 10, 2019
Course Directors:
Instructors:
Dominique Bergmann (Stanford), Rich Carthew (Northwestern), Mathieu Coppey (Inst. Curie), Liam Dolan (Oxford), Kenneth Irvine (Rutgers), Madhav Mani (Northwestern), Adam Martin (MIT), and Max Wilson (UCSB)
Guest Lecturers (partial list):
The summer course is closely linked to the concurrent KITP program Morphogenesis in Animals and Plants: Search for Principles. Course participants will attend the program's daily research seminars as part of the course curriculum. Students and lecturers will also have frequent opportunities for less formal interactions. Confirmed program participants include A. Boudaoud (ENS Lyon), M. Bagnat (Duke), J. Briscoe (Crick Inst.), C. Extavour (Harvard), C. -P. Heisenberg (IST Austria), M. Heisler (U. of Sydney), F. Jülicher (MPI-PKS), T. Lecuit (Collège de France and IBDM Marseille), O. Leyser (Cambridge), E. Meyerowitz (Caltech), D. Montell (UCSB), N. Nakayama (U. of Edinburgh), J. Nemhauser (U. of Washington), A. Roeder (Cornell), E. Sharon (Hebrew U.), S. Shvartsman (Princeton), A. Shyer (Rockefeller), E. Siggia (Rockefeller), X. Trepat (IBEC), and A. Warmflash (Rice).
Course Subject
Morphogenesis is the process by which a single cell transforms into a complex organism. During development, cell growth, division, and differentiation are tightly controlled to form properly shaped, functioning organs. This remarkable control over form, fate, and function is coordinated by a tight interplay of the genetic program of development and physical interactions between cells.
Coordination of physical processes is equally challenging in the development of animals and plants, but the challenge takes different forms on account of the differences in the structure and organization of their cells and tissues. Thus each kingdom developed independent solutions to the same problem of morphogenesis: encoding of shape and structure of a multicellular organism in the genetic code and the process of development. This course will provide a broad and synthetic view of the subject, aiming to distill the general ideas and principles of development from the diversity of morphogenetic processes.
This hands-on research course will integrate laboratory projects and mathematical modeling, aiming to achieve quantitative understanding of specific morphogenetic processes in a variety of model developmental systems including the fruit fly, the liverwort (Marchantia), and tissue culture. It will emphasize quantitative questions in experimental design and new techniques like optogenetics and in toto microscopy, along with advanced image analysis and mathematical model building.
Course Structure
The course is 5 weeks, beginning with a one-week bootcamp, followed by two 2-week lab sessions. Each session will consist of about four experimental projects led by instructors and TAs working closely with groups of 4-5 students. The daily routine involves two morning lectures and discussions, followed by lab work in the afternoons until late in the evenings.
During the bootcamp, students will acquire skills needed to succeed in research projects, including microscopy, quantitative image analysis, and theoretical modeling. There will also be an introduction to model systems of development and laboratory techniques.
The morning lectures will take place in the context of the parallel running KITP program “Morphogenesis in Animals and Plants: Search for Principles”, providing an excellent setting for discussion and exchange with program participants. The scientific agenda of the program aims to closely study morphogenesis in animals and plants, bringing together these diverse communities with experts in physical modeling of morphogenesis.
The program will study how common physical challenges, experienced by animals and plants are solved. Plant and animal lineages diverged before the evolution of multicellularity, strikingly manifested at the molecular level, where each kingdom separately optimized genetic pathways.
Specifically, the program will seek to develop answers towards these questions:
- How are cell behaviors coordinated during development over long ranges?
- How are growing organs divided into distinct zones?
- Are there common organizing principles underpinning regulation of these processes across kingdoms?
- Is there mechanical regulation of growth?
Experimental Projects (partial list)
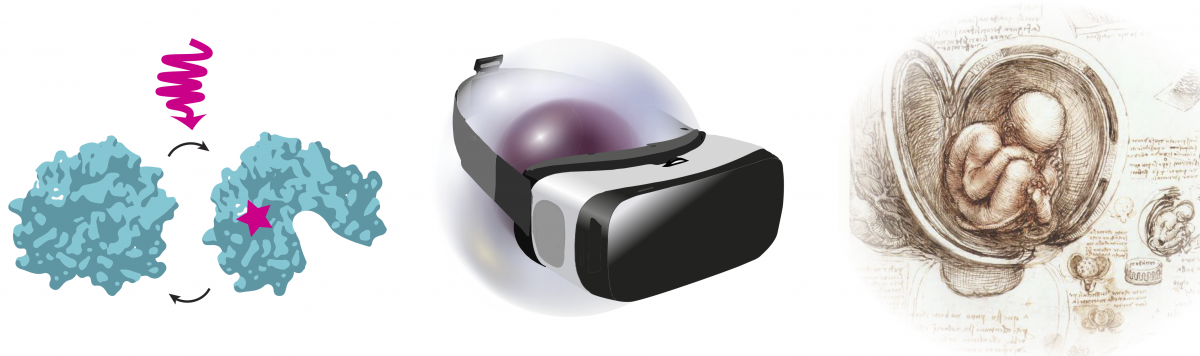
Max Wilson: Virtual morphogenesis using optogenetics
Cells have evolved to survive in fluctuating environments by constantly sensing and responding to external stimuli. Signal transduction pathways are the crucial link through which cells transmit information about their environment to the genome, where they can coordinate a response. In this way, cells create an internal representation of the external world, which they use as the logical basis for executing complex behaviors.
Students will explore the how induced pluripotent stem cells (iPSCs) process spatially and temporally encoded information to make cell fate decisions. They will learn how to engineer light-based control over a number of important signaling pathways in development. They will then be provided with iPSCs in which one of their signaling pathways has been pre-engineered to be controlled with light. Students will spend the rest of the course designing experiments using state-of-the-art light delivery devices to explore a developmental fate choice of interest and developing models to characterize the decisions making apparatus in iPSCs. We hope that students come away from this course with a practical understanding of optogenetics, the various ways in which light can be delivered to cells, and a notion for how signaling information is encoded/decoded by iPSCs.
Dominique Bergmann: Pattern formation in walled cells of plants and algae
Rich Carthew and Madhav Mani: Pattern formation in the Drosophila eye imaginal disc
Mathieu Coppey: Optogenetic control of collective cell migration
Critical events happening during morphogenesis require the collective migration of hundreds or even thousands of cells. Such collective movements depend on two main ingredients. First, physico-chemical signals of large spatial extent, such as morphogenetic gradients or mechanical stresses, inform and guide migration. Second, the communication between cells, via secreted molecules or direct interactions, ensures the coordination and self-organization of the collective migration.
In this experimental project, to evaluate the respective roles of these two ingredients, we propose a quantitative experimental approach based on the light control of long-range guidance cues. The principle will be to actuate collective migration thanks to light patterns shined on engineered cells in culture. Cells will express blue light sensitive molecular systems that can activate intracellular signaling pathways (MAPK and RhoGTPases) known to be involved in collective cell migration. The patterns of light will be generated using a micro-mirror system (DMD) coupled to an epifluorescence microscope. The collective movement will be analyzed either by tracking individual cell trajectories or using image processing methods (PIV, optical flow).
Kenneth Irvine: Three dimensional analysis of morphogenesis in imaginal discs
Adam Martin and Sebastian Streichan: Cellular flow in Drosophila embryo
Stefano Di Talia: Cytoplasmic flows and morphogenesis of early Drosophila embryos
Course Directors:
Course Instructors:
Bill Kristan, Jr. (UCSD), Jimmy Liao (U. of Florida), Matthieu Louis (UCSB), Christopher Potter (Johns Hopkins), Pavan Ramdya (EPFL), Michael Reiser (HHMI-Janelia), Agnese Seminara (U. of Nice), Julie Simpson (UCSB), Marcus Stensmyr (Lund Univ.), Sebastian Streichan (UCSB), Tanya Tabachnik (ZMBBI, Columbia), Massimo Vergassola (UCSD), Daniel Wagenaar (Caltech), David Weisblat (UC Berkeley), and Claire Wyart (ICM Institute)
Guest Lecturers (partial list):
The summer course is closely linked to the concurrent KITP program Neural computations for sensory navigation: mechanisms, models, and biomimetic applications. The course participants will attend the program's daily research seminars as part of the course curriculum. Students and lecturers will also have frequent opportunities for less formal interactions. Confirmed participants include Jelle Atema (Boston U.), William Bialek (Princeton), Dimitry Chklovskii (Flatiron Inst.), Thierry Emonet (Yale), Vivek Jayaraman (HHMI-Janelia), Venkatesh Murthy (Harvard), Katherine Nagel (NYMC), Gonzalo Polavieja (Champalimaud), Sharad Ramanathan (Harvard), Dmitry Rinberg (NYM), Terrence Sejnowski (UCSD), Thomas Shimizu (AMOLF), Nathan Urban (U. of Pittsburgh), Jane Wang (Cornell), and Barbara Webb (Edinburgh U.). A complete list will be available in late March.
Course subject
Physics has become omnipresent in systems neuroscience: it plays a critical role in providing tools to record electrical activity of single neurons, monitor neural activity through multi-photon imaging, and model dynamical behavior of nonlinear systems of interconnected neurons. Neurophysics of Sensory Navigation will equip students with the conceptual and technical frameworks that physics and quantitative biology offer to study the integrated function of neural circuits. The school does not intend to provide a general introduction to systems neuroscience. It aims to be a creative research-oriented course closely linked to the KITP program Neural computations for sensory navigation: mechanisms, models, and biomimetic applications. In addition to their own intensive projects, participants will attend daily seminars of the KITP program, which will provide a broader perspective to the individual research projects conducted in the school.
The course targets graduate students and postdoctoral scholars with some research experience in systems and/or computational neuroscience. The course participants will form a diverse group with different academic backgrounds. We are especially eager to provide this opportunity to scientists from groups underrepresented in the sciences. Our goal is to permit experimentalists to familiarize themselves with theory and advanced data analysis while encouraging theorists to dive into wet-lab experimentation.
Experiments in systems neuroscience require a broad range of techniques, necessitating collaboration between scientists with complimentary areas of expertise. Accordingly, course participants will work in interdisciplinary teams that include both theorists and experimentalists, building common vocabulary and learning the physics and neurobiology that will help them tackle challenging systems neuroscience questions: how do noisy sensors reliably capture multisensory sensory information? How is the integration of sensory signals transformed into decision making? How are behaviors such as locomotion planned and controlled by the motor system? The course will center around research projects covering two themes: (1) mapping and functional characterization of neural circuits and (2) quantitative description and sensory manipulations of elementary behaviors. By working under the guidance of instructors and teaching assistants, students will be encouraged to develop and pursue their own questions.
Experimental Projects (partial list)
Bill Kristan, Jr.; Daniel Wagenaar; and David Weisblat: Tracking, modeling, and electrophysiological characterization of leech scanning behaviors
Most animals scan their environment to search for—among other things—food, potential mates, or places to hide. Leeches are excellent animals for studying scanning strategies because their behavior is robust and their nervous systems are accessible at the level of individual, identified neurons as they behave. Students will characterize scanning behaviors in two different leech species (one is carnivorous, the other sucks blood) to find common elements in leech behaviors as they crawl on substrates of different roughness and follow food clues such as chemical trails and water waves that generate both visual and tactile stimuli. From a detailed analysis of the scanning behaviors, the students will generate models based upon modular behavioral movements. They will then record electrically from identified neurons in the leech central nervous system to seek the neuronal mechanisms underlying different aspects of the scanning behavior. Ideally, these electrophysiological recordings will provide neuronal reality for their behavioral models, which can then be incorporated into biomimetic devices. Figure: time sequence micrographs and tracings of leech body position during a scanning behavior sequence
Pavan Ramdya and Julie Simpson: Creating virtual obstacles to study tactile navigation in adult flies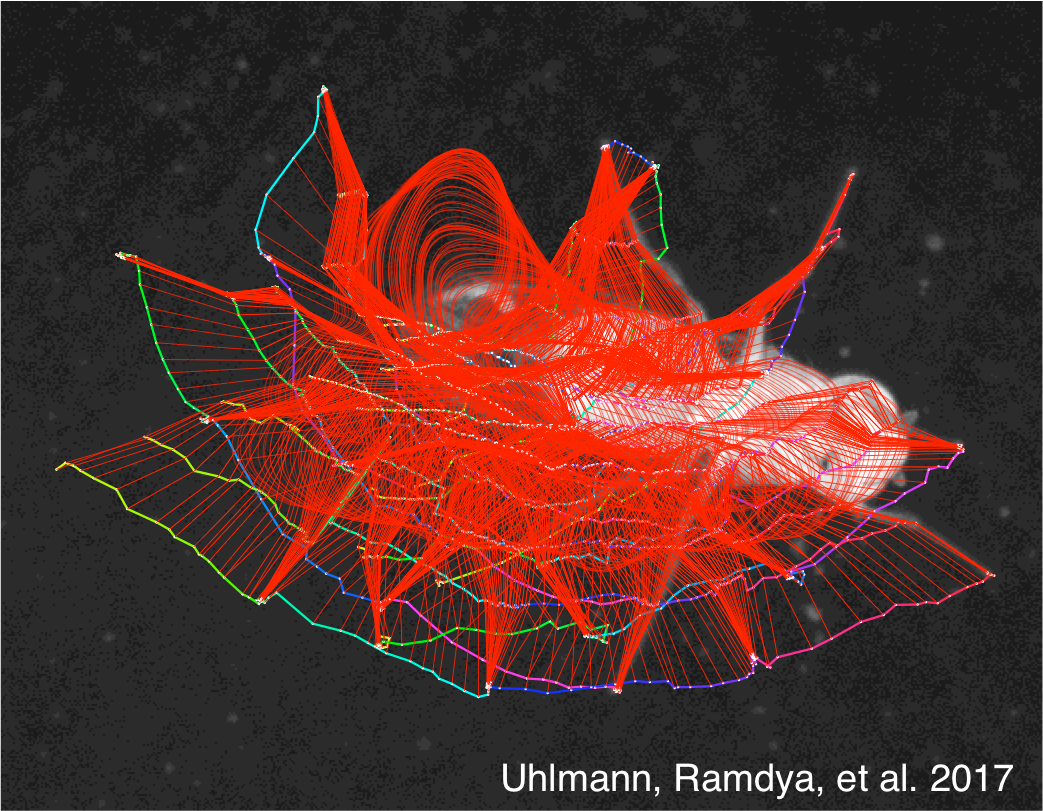
How do insects use mechanosensory information when detecting obstacles and exploring their environment? In this project, we will use limb-tracking software to analyze high-resolution behavioral videos of flies interacting with obstacles in real and optogenetically-generated virtual reality environments to identify the neural algorithms underlying mechanosensory navigation. Figure: Custom software is used to track the body and limb segments of fly while it is walking. This software will be used to quantify limb movements in response to real or virtual environmental obstacles.
Christopher Potter and Marcus Stensmyr: Modeling mosquito navigation behavior in response to humidity and temperature
Mosquitoes depend on temperature and humidity gradients to find suitable locations for egg-laying, to avoid inhospitable climates, and to find humans for a blood-meal. In this module, we investigate the behavioral interactions of adult and larval Aedes aegypti mosquitoes (vectors for Dengue, chikungunya, and Zika viruses) as they explore these crucial physical sensory stimuli. We will generate and test high resolution models linking temperature and moisture gradients with the behavioral movements of mosquitoes and use these to understand the strategies used by these insects to navigate these physical cues.
Matthieu Louis, Agnese Seminara, Sebastian Streichan, and Massimo Vergassola
The Drosophila larva has emerged as a powerful system to dissect the neural basis of sensory-driven behaviors. The larva demonstrates a rich repertoire of orientation responses under the control of a brain composed of fewer than 10,000 neurons that are being mapped in a wiring diagram. We will build an affordable (do-it-yourself) assay to track single larvae and to conduct closed-loop stimulation experiments with light. By optogenetically stimulating the larval olfactory system, we will create virtual olfactory realities. With this technology, we will apply a systems-identification approach to investigate principles underlying the sensorimotor control of odor-driven and light-driven behavior. Using light-sheet microscopy, we will also attempt to monitor the activity of the larval olfactory system during naturalistic food-search behavior. Figure: Remote-control of the trajectory of a Drosophila larva through the optogenetic manipulation of its olfactory system (head position in blue; centroid position in black).
Jimmy Liao and Claire Wyart: The kinematics of zebrafish orientation and navigation
Larvae and juvenile zebrafish exhibit robust orientation behaviors in response to chemical gradients and water flow. In this module, we will study the kinematics of locomotion of individual animals to understand how the initiation and direction of orientation responses is determined by the detection of olfactory cues and both laminar and vortical flows. Building on this analysis, we will examine how the presence of other conspecifics condition the orientation responses displayed by individuals.
Application deadline is March 12, 2017.
Course Directors:
Course Instructors:
R. Dutton (UCSD), D. Huson (U. Tübingen), T. Julou (Biozentrum, Basel), H. McCann (NZIAS), Richard Neher (Biozentrum, Basel), J. Quick (U. Birmingham), Paul Rainey (ESPCI, Paris), Boris Shraiman (KITP, UCSB), E. Toprak (UT Southwestern), E. Wilbanks (UCSB)
Guest Lecturers (partial list):
Course subject
Microbial communities play a profoundly important role on all scales, from carbon cycling in the ocean to cellulose utilisation in the gut of a termite. Microbial communities provide the foundation for ecosystems on the planet and are increasingly recognized as a major factor in human health. While it is convenient to study separate microbial species as they interact with the environment, in reality the environment experienced by any one microbe is shaped by its interaction with other members of the local ecological community. Behavior of an individual cell is intertwined with the behavior of other community members and many of the metabolic functions of a cell can be “outsourced”, making the community the relevant physiological unit. Understanding the function of microbial communities is the current frontier of science at the interface of microbiology with ecology and evolution. What are the “forces” that bind these microbial communities? Is the community more than the sum of its parts? What are the “parts” and how do they interact? How do microbial communities respond and adapt to environmental challenges and how do they evolve? What is the role of horizontal gene transfer? What is the role of competition within a community and how is the diversity maintained?
The course will introduce students to the fundamental questions and to the promising approaches towards the study of microbial communities. Students will get a hands-on introduction to microbial communities in the lab and will gain experience with new technologies for studying microbes. Students will be working in the groups of 4-5 (under the guidance of instructors and TAs) on different projects which will involve microbiology lab work, sequencing, bioinformatic analysis and modeling. Within the framework of the group projects students will have an opportunity to define and pursue their own research questions. A number of short lecture series will introduce basic concepts and new developments. We expect that, even under the constraint of a 4 week course, students will be able to generate interesting “preliminary data” and perhaps make a discovery or two.
The course will run side by side with the KITP workshop Eco-Evolutionary Dynamics in Nature and the Lab. Students will attend the lectures and seminars and will have the opportunity to interact with numerous senior researchers that will be participating in the workshop.
Mini-Courses
- Daniel Huson: Metagenomic sequence analysis (2 lectures + 3h “practical”)
- Eugene Koonin and Richard Neher: Bioinformatics of microbial pan-genome (2 lectures + 3h “practical”)
- Joshua Quick: Nanopore sequencing bootcamp
- Alfred Spormann: Introduction to cellular and community metabolism (3 lectures)
- Joshua Weitz and Forest Rohwer: Quantitative viral ecology (3 lectures)
- Michael Doebeli and Daniel Fisher: Introduction into mathematical modeling of ecological and evolutionary dynamics (2 lectures+3h tutorial)
EXPERIMENTAL PROJECTS (preliminary list)
Rachel Dutton: Microbial communities on cheese
The microbial communities of cheese are relatively simple, easily culturable, rich in species interactions, and undergo reproducible dynamics of community assembly. They are hence ideal systems to study general properties of microbial communities. During the course, we will:
- analyse time series sequence data of community dynamics
- isolate species and sequence and assemble their genomes using NanoPore technology
- study horizontal transfer within cheese communities.
Thomas Julou: Single cell phenotyping to study phage-bacteria interactions
In a complex microbial community, phages, nutrients, toxins, and other parameters are unpredictable. Bacteria respond heterogeneously to changing environments and this heterogeneous response can only be studied at the single cell level. Using a “mother-machine” we will explore single cell responses to fluctuations in nutrients and phage. We will:
- Use the mother machine to study the responses of bacteria to fluctuating nutrients
- Explore dynamics of the interaction between phages and bacteria
- Determine phage life history parameters
Joshua Quick: Nanopore boot camp
Sequencing technology is currently undergoing a second revolution from short-read to long-read sequencing. Nanopore Technologies has developed hand-held sequencers, the MinIon, with very short turn-around times that we will use during the course to sequence. We will:
- use nanopore long read sequencing to assemble genomes from cheese and marine communities
- metagenomic and environmental sequencing
- quantify rearrangements and phage integrations using long reads
Honour McCann: Phylogeography and pangenomics of a kiwifruit canker pandemic
- Use millifluidic technology [see / link to below] to isolate bacteria from leaves and process for sequencing in a single step.
- Engage with new computational approaches for inferring biogeography and evolutionary origins of microbes
- Study the population biology of ICEs
Paul Rainey with Maxime Ardré, Guilhem Dulcier and Laurent Boitard: Millifluidics
- Use MilliDrop technology to isolate leaf colonising bacteria
- Explore population dynamics of siderophore producers / non-producers
- Analyse interactions between community members
- Perform statistical analysis on population data using custom-built pipelines.
Paul Rainey with Steven Quistad: Phage and the evolution of microbial communities
- Isolate and characterise by DNA sequencing community members (phage and bacteria).
- Determine the effect of selection and lateral gene transfer on the evolution of communities via phenotypic assay.
- Delve into the dirty depths of DNA data and discover promiscuous phage, malicious microbes and culturable obscurities.
Erdal Toprak: Building and running a morbidostat
A morbidostat is a versatile computer controlled continuous culture device that adjusts the growth environment to maintain a specified growth rate, for example by adjusting the antibiotic concentration in the medium. We will:
- build a morbidostat device
- morbidostat experiments to study evolution of antibiotic resistance
- analyse time series data from morbidostat experiments by phenotyping and genotyping evolved populations
Lizzy Wilbanks: Pink berry microbial consortia
The pink berries are photosynthetic microbial aggregates composed primarily of two closely associated species sulfur-metabolizing bacteria. These species carry out a tight-coupled, syntrophic sulfur cycle within these macroscopic aggregates that found at the sediment-water interface of intertidal pools in the Sippewissett salt marsh (Falmouth, MA). During the course, we will:
- compare different long-read data for analysis and assembly of metagenomes
- analyze strain-level biogeography between aggregates and along spatial transect
- investigate the phages associated with pink berries and immunity profiles of different isolates.
Course Directors:
Course Instructors:
S. Eaton (MPI-CBG, Dresden), C. Extavour (Harvard), N. Goehring (MRC LMCB, London), P. Lemaire (CRBM, Montpelier), M. Mani (NWU), A. Oates (MRC LMCB, London), A. Pavlopoulos (Janelia, HHMI), B. Shraiman (KITP, UCSB), S. Streichan (KITP, UCSB), A. Warmflash (Rice) and E. Wieschaus (Princeton)
Guest Lecturers (partial list):
Course subject
This course aims at bringing together the Biology and Physics perspectives on Morphogenesis - the process through which animals and plants acquire their physical form and biological function. Morphogenesis is a developmental program encoded in DNA and kick-started by “maternal factors” in the egg. Developmental Biology has made enormous progress in identifying the genes that specify developmental axes and the general body-plan as well as the numerous genes and biochemical signals that control growth and cell differentiation. Yet, even as we know the expression of what gene would cause a fruit-fly to grow a leg on its head instead of an antennae, we have little understanding of how controlled growth and cell differentiation actually generates the distinct shape and structure that make a leg rather than an antennae. A century ago, before the advent of developmental genetics, D’Arcy Thompson viewed Morphogenesis as an essentially physical process of controlled growth. Revisiting Thomson’s agenda, the challenge is to connect the macroscopic dynamics of morphogenesis to the underlying molecular genetic and cell-biological processes. Physics perspective in particular focuses on the dynamical aspect of morphogenesis. To use a metaphor, what are the “laws of motion” that define the developmental trajectory from maternal factors as “initial conditions” to embryonic structures and beyond? Physics perspective also brings to fore the role of intercellular interactions in coordinating development and, in particular, the role of mechanics alongside with biochemical signals governing collective and individual behavior of cells in tissues.
The course will be anchored by laboratory projects involving different morphogenetic processes in model organisms such as the fruit fly Drosophila melanogaster, the nematode Caenorhabditis elegans, zebra fish (D. rerio), a sea squirt (Ciona) and a crustacean (Parhyale hawaiensis). Experimental projects will aim to introduce quantitative approaches to the study of the dynamics of morphogenesis.
Course structure
This five-week course will consist of a first "bootcamp" week to be followed by two 2-week research project sessions. Each session will consist of three or four experimental projects led by instructors and TAs who will closely work with groups of 4-5 students. Each day will consist of a morning lecture and discussions followed by lab work late into the evening.
The bootcamp week will be aimed at introducing all students to a basic set of microscopy, quantitative image analysis and theoretical modeling tools that will be necessary for the later research projects. Bootcamp will also introduce students to different laboratory model systems of development.
The course will run side by side (and share review lectures and research seminars) with the KITP workshop "From Genes to Growth and Form", which will provide a fertile forum for discussions and the exchange of ideas with the workshop participants. The scientific program of this workshop will be anchored by the following intersecting themes, which are central to the subject of the course:
Role of time in patterning space: will aim to reexamine the scenario of static spatial patterning by a gradient of morphogen concentration confronting it with the observations of an adaptive response and the hypothesis that cells respond only to the time derivative.
Interplay of global and local patterning signals: will focus on the relation between local polarization of cell and global anisotropy; examine the extent of global planar cell polarity order in tissues; reconcile observed local cell deformation with global distribution of mechanical stress.
Mechanical regulation of growth: consolidate evidence for mechanical regulation of growth of tissues in different systems. Evaluate different approaches to measuring stress in live tissues and monitoring its dynamics. What do we know about the molecular pathways of mechano-regulation?
Cellular “flows” and their generation: How can we determine the driving forces behind observed cellular flows? Comparison of cell-deformation, cell intercalation and cell proliferation as the drivers of global cell rearrangement.
Polarity and anisotropy in defining tissue morphology: Consolidate existing knowledge on the mechanisms of planar cell polarity (PCP and Fat/Ds pathways) and their role in defining clonal shapes and tissue anisotropy and controlling cellular flows and tissue rearrangement.
In toto organogenesis: will focus on the few model morphogenetic system, like Drosophila ovarian development, where the dynamics of morphogenesis could be examined in its entirety.
Comparative morphogenesis: will examine the similarity and differences between developmental mechanisms in different limbs and organs, e.g. wing vs leg and eye of Drosophila, and in different animals e.g. Drosophila vs wasp or beetle.
EXPERIMENTAL PROJECTS (preliminary list)
Suzanne Eaton: Drosophila wing development
Nathan Goehring: C. elegans embryonic development
Patrick Lemaire: Ciona embryonic development
Andy Oates: Zebra fish somitogenesis
Aryeh Warmflash: Stem cell differentiation patterns in vitro
Anastasios Pavlopoulos: Developmental morphogenesis in a crustacean
Sebastian Streichan and Eric Wieschaus: Cellular flow in Drosophila embryo
Application deadline is March 1, 2015.
Course Directors
Course Instructors
Mónica Bettencourt-Dias (Inst. Gulbenkian de Ciência), Cassandra Extavour (Harvard University), Michael Lynch (Indiana University), Jitu Mayor (NCBS Bangalore), Daniel Needleman (Harvard University), and José Pereira-Leal (Inst. Gulbenkian de Ciência)
Guest Lecturers (partial list)
Course subject
Recent years have witnessed a revolution in our ability to quantitatively query and manipulate biological systems. Live imaging and genome editing techniques combined with quantitative approaches have made it possible to uncover the molecular basis of many cellular processes. Indeed, cell biology has made tremendous progress towards revealing the molecular mechanisms involved in a plethora of processes ranging from cellular maintenance to the coordinated cellular decisions involved in embryonic development. However, these studies on how cellular functions are realized have been mostly devoid of the question of how these same functions came to be through evolution and natural selection.
The quantitative study of evolution, on the other hand, has been primarily focused on a description of evolutionary dynamics at the scale of populations. Here, the evolutionary history of species in a population is quantified based on the natural selection afforded by pressures such as competition with other species and changing environmental conditions. These studies focus mostly on the effect of mutations on fitness but not on how these mutations, through their effect on cellular function, lead to a reshaping of evolutionary landscapes.
A gap has existed between cell and evolutionary biology. Namely, the uncovering of how novel cell biological function evolves as organisms adapt to different environmental conditions. The time is ripe to bridge this gap as the technology that has allowed for the cell biology revolution in model organisms can now be applied in the context of their fully sequenced non-model organism counterparts. The aim of this course is to make progress towards filling this gap by focusing on the evolution of cellular processes ranging from horizontal gene transfer, to the evolution of intracellular functions such as trafficking and mitosis, to the evolution of embryonic developmental programs. The course will combine state-of-the-art in vivo microscopy, bioinformatics analysis and theoretical modelling in a plethora of model and non-model systems aimed at uncovering the evolutionary basis of cellular function and behavior at these multiple scales.
Course structure
This five week course will consist of a first "bootcamp" week to be followed by two 2-week research project sessions. Each session will consist of three or four experimental threads led by instructors and TAs who will closely work with groups of 4-5 students. Each day will consist of a morning lecture and discussions followed by lab work late into the evening.
The bootcamp week will be aimed at introducing all students to a basic set of microscopy, quantitative image analysis using Matlab and theoretical modeling that will be necessary for the later research projects. The lectures will take place in parallel with the KITP workshop "Evolutionary Cell Biology" providing a fertile forum for discussions and the exchange of ideas with the workshop participants.
EXPERIMENTAL PROJECTS (preliminary)
The Evolutionary Cell Biology of Gene Transfer. Instructor: Rob Phillips.
Microbial populations live in a fertile soup of foreign genetic information. Whether by viral infections, conjugative transfer, or direct uptake of the DNA through transformation, bacteria frequently share genetic information with their neighbors through horizontal gene transfer. Despite its deep evolutionary importance, the mechanistic details about how such gene transfer works (both at the molecular and systematic level) remain enigmatic. In our laboratory rotation, we will use microscopy to systematically explore how bacteria take up DNA. By using fluorescently labeled proteins that bind to specific sequences on the transferred DNA, we will develop a real-time picture of DNA uptake and dynamics and consider its effect on evolution across many timescales.
Vesicle Transport Evolution in the Ciliated Protozoan Paramecium. Instructor: Michael Lynch.
In eukaryotic membrane trafficking, both soluble cargo and membrane-bound components must travel efficiently and specifically between organelles via lipid-bound vesicles. These vesicles are directed specifically to their target membranes via sets of interacting protein determinants that are themselves tightly controlled in space and time. These protein determinants are members of large gene families, whose duplication and diversification have enabled trafficking pathways in different eukaryotic lineages to diversify and take on new roles. We will focus on one trafficking pathway, endocytosis, whose associated protein determinants have shown remarkable plasticity over evolutionary time. We will identify the molecular determinants involved in one endocytic trafficking step, trace their evolutionary relationships, and then track them at the subcellular level. We will look particularly for instances of functional change, or hints of functional diversification occurring in these determinant subfamilies, and test our hypotheses of functional change gleaned from targeted sequence analysis.
This work will employ members of the Paramecium “aurelia” complex, a cryptic species complex of ciliates that diverged several hundreds of millions of years ago following two complete genome duplications. As outgroup comparators, several pre-duplication species are available. Having a large macronucleus, ciliates are uniquely amenable to rapid transformation by injection of chromosomal constructs with key genes fused to GFP, which can then be used to visualize subcellular localizations of gene products.
The evolution of gene regulation and pattern formation in development. Instructor: Hernan Garcia.
The evolution of embryonic development is the substrate for the emergence of new body plans. The general features of these developmental programs are highly conserved between closely-related species. Indeed, in the Drosophila family, of which the fruit fly D. melanogaster is its most prominent member, the same transcription factors lead to the expression of genes in similar patterns in the early embryo. Despite phenotypic similarities in developmental programs, homologous regulatory decisions are realized by different arrangements of binding sites for repressors and activators in each species. For example, the regulatory region leading to the famed stripe two of the eve gene has endured significant rearrangements as binding sites have been deleted, appeared or changed in their affinity and in their spacing. Still, all of these regulatory regions lead to stripes that are qualitatively comparable and that can even rescue development when inserted into different species.
Using novel imaging techniques that allow for the quantification of transcription in single cells in living embryos we will determine how different species create the “same” pattern of gene expression. We will insert regulatory regions for the eve stripe 2 from different species as well as proposed ancestral reconstructions into D. melanogaster and some of its allied species. These experiments will open the door to determining the role of regulatory drift in these highly conserved programs as well as generate a “phylogeny of strategies for pattern formation”. This phylogeny will then be compared to the more familiar phylogeny of the DNA regulatory sequence in order to develop theoretical models of how regulatory architecture determines function and how both evolve in tandem. Finally, in order to explore the limits of what evolution could have created we will use synthetic biology to rewire this network by creating the same stripe in response to engineered, exogenous transcription factors and comparing the dynamics of formation of this genetic rescue to those dynamics of the wild-type stripes.
Evolution of pattern formation strategies. (A) Different fly species realize a stripe of expression of the same gene by using different regulatory architectures. Namely, the placement, number and affinity for activators and repressors in the vicinity of the gene. (B) Live imaging techniques give access to the dynamics of stripe formation in different species and to contrasting this “phylogeny of pattern formation strategies” to the more familiar DNA sequence phylogeny.
The evolution of an active membrane composite. Instructor: Jitu Mayor with Thomas van Zanten
The outer membrane of the living cell is the interface that demarcates the cell and its environment. Its chemistry is largely build up from lipids and proteins. Local organization of this chemistry primes the cell to adapt and react to the external milieu and communicate information about its internal state. In a range of metazoan cells the membrane organization is not simply passively constituted, but it is actively maintained away from thermodynamic equilibrium. This characteristic emerges from an interplay between the constituents of the membrane closely juxtaposed to a dynamic actin-based cortical layer, together forming an active composite. Cells consume energy to mechanically restructure this composite that consists out of, amongst others, the evolutionary conserved proteins such as actin, actin binding proteins and myosins. Perturbing the non-equilibrium state of cells has a dramatic effect on essential functions such adhesion or endocytosis.
Do the different cellular organisms we find in the local marine or soil environment display an active organised plasma membrane? Using an experimental scheme that was originally used for studying the membranes of metazoan cells we will explore the organisational capacity of membranes across the phylogenetic tree. This approach will consist of doping the membranes of protists with fluorescence lipid dyes and imaging FRET between the doped lipid probes to evaluate their local organization at the nanometer scale. Another way to explore this question is take a more bio-informatics approach and trace back different proteins over evolutionary time using available genome data.
Combined projects of Monica Bettencourt-Dias, Cassandra Extavour, Jose Pereira Leal, and Dan Needleman.
Within-Species Variation in Cell Biology in Drosophila
Everyone is unique. We have different appearances, different behaviors, and different talents. But to what extent are the dynamics and organization of our cells different? The answer is currently unknown. Variations in cellular behaviors between different people likely underlie many of our obvious external differences and might be responsible for differences in susceptibility to disease. Understanding the extent and nature of the genetic basis of within species variations in cell biology will also help to understand the evolution of variations in cell biology.
Recent work in the nematode Caenorhabditis elegans has shown that there is extensive within-species variation for the structure and dynamics of the mitotic spindle. We will extend this work by investigating the extent of within-species variation for a variety of cell biological features in the fruit fly Drosophila melanogaster. We will use quantitative approaches to characterize this variation and we will attempt to map the genetic basis of any differences we discover.
Between-Species Variation in Cell Biology in Insects
Above is a “t-projection” of a 26-hour whole Gryllus (cricket) embryo time lapse of the H2B-GFP cricket transgenic line, taken from 5 to 31 hours after egg laying (AEL). Each time point is colored in a different color along a gradient lookup table and then stacked (with dark warm colors at the beginning and yellow to white at the end of the time lapse). This shows the general course of the time lapse. (Taro Nakamura & Seth Donoughe)
Drosophila melanogaster has served as the exemplar for molecular and quantitative understanding of insect development for nearly half a century. However, this fly belongs to the lineage furthest derived from the last common insect ancestor, and its developmental and cell biological characteristics are unlikely to be representative of the most species-rich animal group, the insects. Working with not only Drosophila, but also the beetle Tribolium castaneum and the cricket Gryllus bimaculatus, students will be able to perform cell biological comparisons between lineages that diverged hundreds of millions of years ago, yet still share a highly conserved adult body plan.
Because early insect embryos undergo syncytial cleavage, in which nuclear cleavage takes place without cytokinesis, these embryos are essentially huge multinucleate cells. Moreover, although all of these insects reproduce sexually, their haploid, unfertilized eggs can be induced to begin cleavage and start to proceed through development. This provides an opportunity to compare the dynamics of nuclear spacing, synchrony and number of nuclear divisions, sexual versus asexual development, and nuclear dynamics across a broad phylogenetic range.
For both Tribolium and Gryllus, we will provide stable transgenic lines expressing fluorescently labeled cell components, enabling live imaging and quantitative analysis of image data. In addition, we will be able to manipulate embryogenesis in these animals by injecting mRNAs encoding proteins of interest, including fluorescent tags for cell biological components, pharmacological agents, and inducing RNA interference against genes of interest.
A zoomed in view of nuclei at the mid-blastoderm stage of Gryllus, with four successive time points 10 minutes apart superimposed in different colors. For some nuclei, there is no overlap between neighboring time points. (Seth Donoughe)
Visualization of centrosome duplication using labelled tubulin in embryos.
Cellular Evolution In Cancer- Does It Provide New Therapeutic Opportunities?
Cancer cells show several modifications in cellular characteristics, that allow them to survive, proliferate and conquer new environments. Those modifications are often specific of cancer cells and can be used for selectively killing them.
The centrosome, the major microtubule-organizing center in animal cells, participates in processes such as cell division, migration and establishment of cell polarity. Centrosome aberrations are commonly observed in cancer. Those changes might lead to increased genomic diversity and ability to invade and conquer new environments. However, those changes can also lead to very abnormal divisions that result in cell death. Mechanisms that allow cancer cells to survive in the presence of centrosome aberrations have been recently described and are being used to selectively kill cancer.
We have recently conducted a phenotypic screen for centrosome changes in a panel of 60 human cell lines that reflect different tumor types. We have seen that centrosome aberrations are a hallmark of cancer cells. While there are a few major mechanisms used to cope with those changes, we have observed that cancer cells “are creative” and can adapt in multiple ways. We will extend this work by investigating the strategies cancer cells use to adapt to centrosome changes. We will do so by using quantitative analyses of cell cycle progression and mitotic division, while monitoring chromosomes, mitotic spindle and centrosomes in live cell populations. Information on gene expression and proteome is available for the different cell lines, so ultimately cellular mechanisms might be linked to molecules. We can further investigate whether mechanisms of adaptation are already present within the population as standing variation, or arise after the appearance of centrosome changes. We will do that by acutely manipulating centrosome and spindle structural properties in normal and cancer cells, and investigating how they adapt to them.
We can also model those processes to predict whether drugs that target them would be effective in eliminating cancer cells. This project will involve microscopy, tissue culture, genetic manipulation of cells, quantitative imaging, bionformatic analysis and modeling.
Natural variation in the cell biology of marine micro-organisms
Life started in the sea, and the sea is still a source of breathtaking cellular diversity. This includes serial endosymbiotic systems where we find stable associations of cells nested within cells. For example, some dinoflagelates are found in algal blooms, where they co-exist alongside cells that have a silica-based cell-wall with amazing diversity and beauty under the microscope. The two types of organisms are examples of microalgae, primary biomass and oxygen producers in the ocean. They include many toxic species that experience explosive growths, giving rise to algal blooms that cause untold economic costs to a variety of industries worldwide, for example including shellfish farming and fisheries.
Marine micro-organisms are a good system to study natural variation of cells “in the wild,” particularly algal blooms where high concentration of diverse species co-exist. While a lot of attention has been devoted to inter-species variation and to species composition of marine micro-algae, little attention has been devoted to within-species variation. For example, do all individuals in a population for a given species multiply at the same rate? This is an important cell biology question with an impact in the dynamic of algal blooms.
In this project we will sample the local marine environment and characterize its species diversity using different techniques. We will isolate species and expand them in culture for cell biological characterization, studying natural variation of division rates, cell size, etc. We will pay particular attention to characterizing microtubule-derived organelles and structures, such as centrosomes and mitotic spindles, as proposed in the other projects in this session.
We will also explore the genetic variation and the basis of phenotypic variation using a genomics and bioinformatics approach. We will analyze publicly available genome sequence data, using bioinformatics and molecular evolution approaches, to detect for signatures of selection, expansion of protein families, variation of biophysical properties of proteins etc., in relation to phenotypic variation.
Students will be able to choose to focus more on the computational or on the wet-lab side of the project, or a hybrid of both.
Microbial Strategies for Survival and Evolution
Course Directors:
Course Instructors:
Guest Lecturers (partial list):
COURSE SUBJECT
Microbial life is considerably more complex than is commonly appreciated. Far from merely proliferating exponentially until nutrients are exhausted, microbes engage in a wide range of adaptive behaviors. For example, the diauxie phenomenon associated with the nutrient induced shift of bacterial metabolism was at the heart of Monod and Jacob’s discovery of regulated gene expression. Much like cells in the multicellular organisms, microbes – bacteria and yeast for the purposes of this course – can exist in different phenotypic states. Microbes can switch between different phenotypes and the phenotypic states can persist through cell division thanks to the existence of epigenetic memory. The mechanisms of the epigenetic memory are remarkably diverse, from hysteretic transcriptional regulation circuits to metabolic circuits to DNA methylation and are a subject of much current research, focusing in particular on the role of inductive signals and stochastic fluctuations in switching between states. Interestingly, epigenetic switching coexists with mechanisms of genetic switching via genomic rearrangement (phase variation) or recurrent mutations bridging in a very interesting way the phenotypic dynamics of microbial cells and evolutionary dynamics of microbial colonies. Quantitative exploration of these phenomena will be the central themes of the course.
EXPERIMENTAL PROJECTS (preliminary)
Bet-hedging and rapid phenotypic switching
Instructors: Paul Rainey and Silvia De Monte
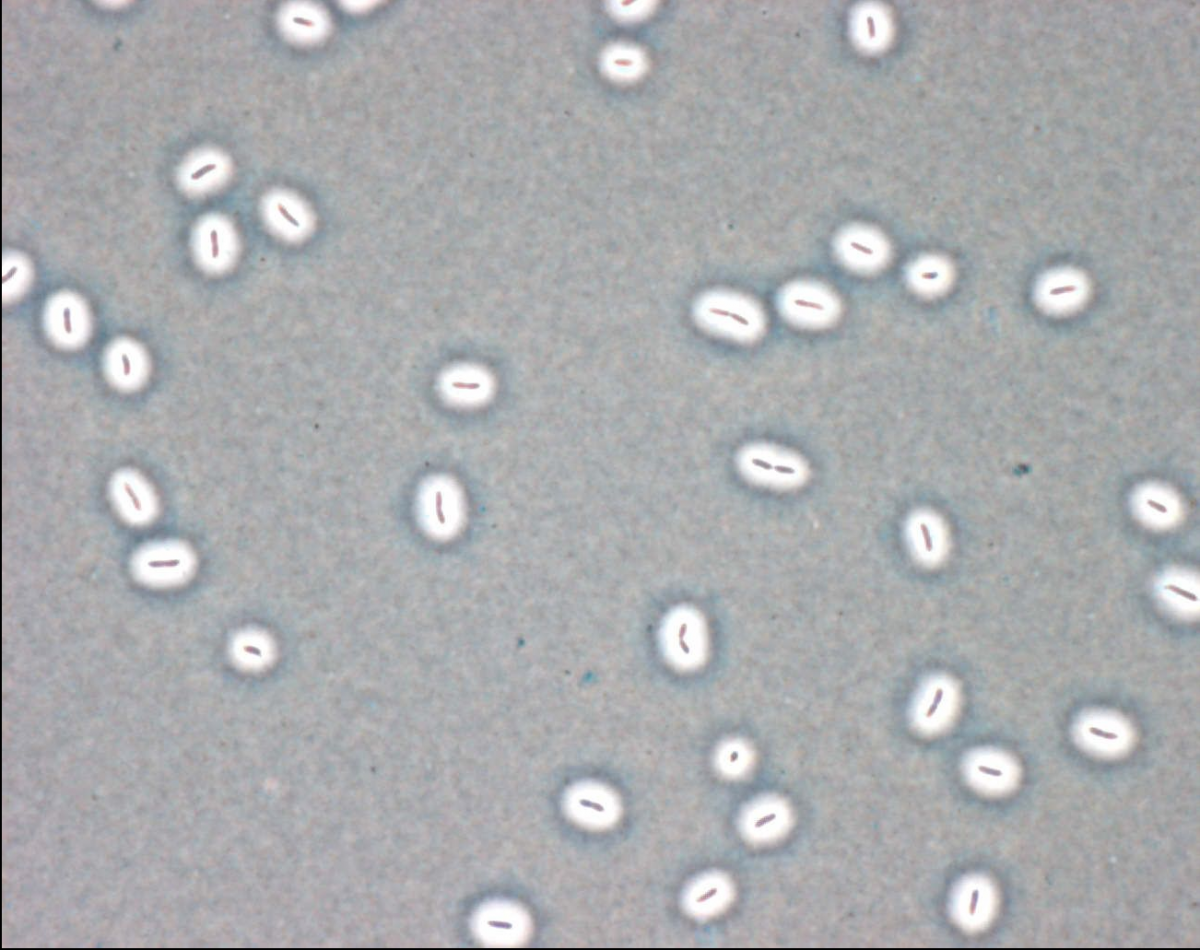
Mutational and non mutational resistance to bacteriophage.
Instructor: Josep Casadesús
Classical studies showed that bacterial resistance to phages can be acquired by mutation. In certain cases, however, bacteriophage resistance can be also acquired by non-mutational mechanisms. For instance, the Salmonella enterica opvAB operon encodes cytoplasmic membrane proteins that alter lipopolysaccharide O antigen chain length, and confer resistance to bacteriophage P22. Because expression of opvAB undergoes phase variation, S. enterica populations contain a mixture of opvAB-ON and opvAB-OFF cells. Infection of Salmonella enterica with the virulent phage P22 H5 kills the major opvAB-OFF subpopulation and selects the opvAB-ON subpopulation. Cessation of phage challenge permits the appearance of a novel opvAB-OFF subpopulation generated by opvAB phase variation. Epigenetic resistance thus pre-adapts S. enterica to survive phage challenge in a reversible manner and without the fitness costs associated with mutation. However, the S. enterica opvAB-ON subpopulation is avirulent in mice and unable to proliferate in macrophages. Phase variation of opvAB expression may thus be viewed as a tradeoff between virulence and phage resistance. We will characterize phage and bacterial populations using fluctuation tests, assays of fitness costs associated with individual mutants and flow cytometry to determine rates of switching between ON and OFF states.
____________________________________________________________________________________
Quantitative study of “persistor” behavior
Instructor: Nathalie Questembert-Balaban
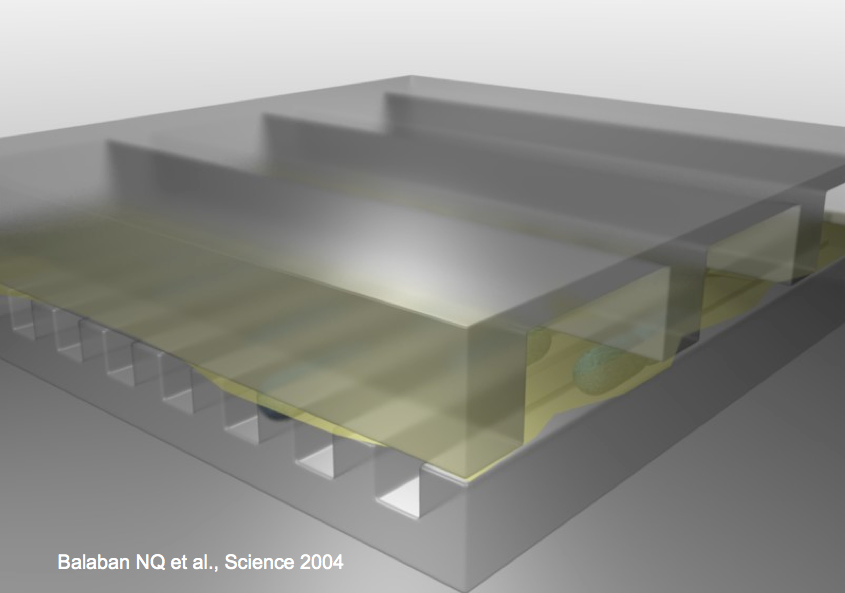
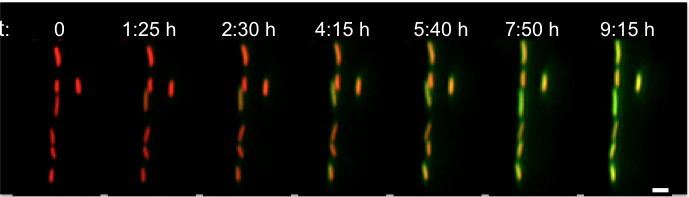
Stochasticity in phenotypic switching: Stochastic Pulse Regulation in Bacterial Stress Response
Instructor: Michael Elowitz
Gene regulatory circuits can use dynamic, and even stochastic, strategies to respond to environmental conditions. Using individual Bacillus subtilis cells we will explore the general stress response mediated by alternative sigma factors and characterize effects due to energy stress. Models based on minimal genetic circuitries will be constructed to which data from single cell dynamics will be fitted. The overall goal is to understand how how bacteria encode signals using stochastic pulse frequency modulation through a compact regulatory architecture.
SigmaB activation, from Locke et al, Stochastic Pulse Regulation in Bacterial Stress Response, Science 2011: Vol. 334 no. 6054 pp. 366-369
To watch a movie see http://www.elowitz.caltech.edu/graphics/1208144s1.mov
____________________________________________________________________________________
Evolution of cooperation in S. cerevisiae
Instructor: Andrew Murray
Recent experimental evolution using yeast resulted two phenotypes that depend on cells sticking together. One is the formation of multicellular clumps to successfully exploit the nutrients harvested by a secreted common good and the other is a circadian oscillator that is detected by its ability to regulate intercellular adhesion. Students will study these
strains to try and understand the mechanisms that regulate clump size. In particular we will ask how the nutrient-harvesting clumps control their size: whether connections between cells break at random or whether the probability of breaking increases with age or with clump size. Models will be developed to predict the distribution of clump sizes. For one of the nutrient-harvesting strains clump size varies depending on the nutrient it receives: wewill determine how this control works. We will also analyze the oscillator. This type requires precise temporal control of cellular adhesion. We will determine whether it is possible to monitor adhesion directly and perform experiments to unravel causal factors.
Clumpiness in the evolved clone of S. cerevisiae due to failure to separate (see Koschwanez et al eLife 2, pp. e00367 (2013)
____________________________________________________________________________________
Rapid evolution in bacterial contact inhibition system
Instructors: Sanna Koskiniemi, Christopher Hayes and David Low
Contact-dependent growth inhibition (CDI) is a phenomenon in which one bacterium inhibits the growth of another upon direct cell-to-cell contact. During CDI, a large stick like exoprotein known as CdiA with a highly divergent C-terminal end containing the toxin domain is transported to the inhibitor cell surface. Upon cell-to-cell contact the toxic C-terminus is delivered to target cells. To protect inhibition from self, a small CdiI protein binds specifically to its cognate toxin and prevents inhibition. The interactions between inhibitor and target cells are intricate and delivery of toxin to target cells requires multiple steps, including binding of a receptor on target cell surface, transfer through the inner and outer-membrane as well as binding of the toxin to an intra-cellular protein required for toxic activity. Disruption in any of these steps allows the target cells to survive and thus resistance development should occur frequently. However, the protein players identified so far consist mostly of essential proteins where even small substitutions might have large detrimental effects on the cell fitness. We have recently carried out a long-term evolution experiment using Salmonella in which derived bacteria evolved the capacity to contact-inhibit the ancestral type. We will explore the adaptive significance of this behavior through construction of simple models and will subject strains from the experiment to genetic (genomic) and phenotypic analyses in order to understand the basis of derived contact-dependent killing of ancestral types.
For additional background please see Hayes et al, Cold Spring Harb Perspect Med 2014
Course Directors:
Thomas Lecuit (IBDML, Marseille), Joel Rothman (MCDB, UCSB), Ewa Paluch (MRC LMCB, UCL London), Boris Shraiman (KITP, UCSB).
Course Instructors:
Otger Campas (UCSB), Thomas Gregor (Princeton U), Lars Hufnagel (EMBL Heidelberg), Denise Montell (UCSB), Pierre Neveu (EMBL Heidelberg), Bill Smith (UCSB)
Guest Lecturers (partial list):
D. Axelrod (Palo Alto), B.Baum (London), Y. Bellaiche (Paris), J. Briscoe (London), A. Boudaoud (Lyon), E. Davidson (Pasadena), S. Eaton (Dresden), M. Gonzalez-Gaitan (Geneva), S. Grill (Dresden), CP. Heisenberg (Vienna), K. Irvine (New Brunswick), F. Julicher (Dresden), A. Maddox (Montreal), E. Munro (Chicago), J. Nelson (Palo Alto), A. Oates (London), K. Oegema (San Diego), P. O’Farrell (San Francisco), O. Pourquie (Strasbourg), J. Prost (Paris), E. Siggia (New York), D. Sprinzak (Tel-Aviv), J. Spudich (Palo Alto), D. Strutt (Sheffield), J-P. Vincent (London), E. Wieschaus (Princeton).
Course Subject:
This Course will focus on the fundamental problem of understanding the dynamics of morphogenesis: the process that converts the genetic blueprint of a multicellular organism into the complex physical structure. How does the morphogenetic "program" of animal development convert genetic information into shape, form and function? To answer this question one needs to understand the dynamics of controlled growth and differentiation, which is directed by intercellular signals and unfolds on the mesoscopic scale of growing tissues. Morphogenesis involves numerous interacting processes that result in phenotypes of great complexity, disentanging which requires quantitative models and quantitative measurements. Thus the goal of the Course is to advance the D'Arcy Thompson's agenda of quantitative description of "Growth and Form" using the full power of modern imaging and molecular genetics which makes the field ready for rapid progress.
On the technical level, the course will introduce several model organisms including D. melanogaster, C. elegans and Ciona and provide instruction on live imaging, micro-manipulation, and genetic and chemical perturbations as quantitative tools to study developmental dynamics. Experimental work will be complemented by theoretical and computational modeling and analysis and involve collaboration with the participants of the concurrent KITP workshop on "New Quantitative Approaches to Morphogenesis".
Course structure:
The 5 week Course will provide an intensive laboratory experience, involving research level projects in small teams of "students" guided by Instructors and TAs. In addition the students will attend and participate in research seminars at the KITP workshop on "New Quantitative Approaches to Morphogenesis".
|
Monday |
Tuesday |
Wednesday |
Thursday |
Friday |
Saturday |
Week 1 Boot-camp |
Safety training Introduction to model organisms |
Introduction to model organisms |
Introduction to model organisms |
Introduction to model organisms |
Principles of microscopy. Demos |
Image processing: Ideas and tools |
Week 2 1st project Week 4 2nd project |
Supervised experiments |
Supervised experiments |
Supervised experiments |
Supervised experiments |
Supervised experiments |
Supervised experiments |
Week 3 1st project Week 5 2nd project |
Data Analysis/ Additional experiments/ Modeling |
Analysis/ Additional experiments/ Modeling |
Analysis/ Additional experiments Modeling |
Analysis/ Additional experiments / Modeling |
Analysis/ Additional experiments/ Modeling |
Project presentations |
Boot-camp: Daily schedule:
9:30am-noon: |
2 tutorial lectures |
1pm – 6pm: |
Demonstrations/basic lab skills development |
Project Sessions: Daily schedule:
9:30am-noon: |
Lectures/discussions at KITP |
12-1pm |
Lunch |
1pm – 6:30pm |
Project supervision; Students divided into three groups (~ 4 per group) working in parallel with instructor teams. |
6:30-8pm: |
Dinner (UCSB dining services) |
8pm-midnight: |
Open Lab time |
Sunday Social activities: Beach BBQ, whale-watching, hiking, sports activities.
Topics covered by the boot-camp:
- Introduction to model organisms (Drosophila, C. elegans, Ciona, etc.) and system-specific tools (basic genetics; developmental characteristics; sample preparation)
- Principles of microscopy. Basics optics and theory and practice of biphotonics
- Image segmentation, particle tracking; optical flow and PIV.
Preliminary description of specific projects :
Project 1: Quantifying transcription and pattern formation in developing fly embryos (Thomas Gregor, Princeton). The pattern blueprint of the adult fly is determined by only a handful of genes within the first 3 hours of embryogenesis. Qualitatively, this system is extremely well understood, and is amenable to quantitative analysis leading to a comprehensive mathematical description of early transcription and patterning processes. We propose two specific goals: 1) live imaging and modeling of gene expression patterns, and 2) measurement and modeling of gene regulation at the single molecule level. For 1) we have transgenic fly constructs that express GFP fusions with various genes of the early patterning cascade, and propose to image, quantify, and model their spatio-temporal dynamics by confocal microscopy. For 2) we will follow transgenic fly constructs expressing alterations of a specific enhancer element that is crucial for early patterning. Using a novel mRNA labeling technique that allows us to optically count individual mRNA molecules in whole embryos, we propose to quantify the effect of the alterations and use stochastic models of transcription to study structure-function relationships of this enhancer element.
Project (2) Building a Single Plane Illumination Microscope (SPIM). (Lars Hufnagel, EMBL). Embryonic development is a highly dynamic process involving many spatial and temporal scales. Novel light microscopy methods are needed to elucidate fundamental morphological processes and to enable close contact with theoretical modeling. The light-sheet concept has proven to be high suitable to image developmental processes. During the 1st week of this project, students will assemble a light-sheet microscope on an optical table. Students will receive hands-on training in basic optics concepts and learn how to program the microscope control software in LabView. During the 2nd week they will quantify/calibrate optical properties of the setup by imaging fluorescent beads (2 days). We will teach students (2 days) light-sheet microscopy specific mounting, image processing and analysis techniques, image stack fusion algorithms and de-convolution, and image the dynamics of (GFP-labeled) nuclei in a Drosophila syncytial blastoderm.
Project (3) SPIM imaging and morphometric analysis of marine invertebrates (Bill Smith, UCSB, and L. Hufnagel, EMBL). Embryos of marine invertebrates such as ascidians and sea urchins, are attractive models for studying developmental dynamics owing to their transparency and ease of culture. This project will avail of the world-class marine laboratory at UCSB and the SPIM built in Project (2). Expression constructs driving GFP fusion proteins of morphogenetically important proteins will be introduced into embryos by microinjection or electroporation. Using image analysis tools we will focus on quantifying and correlating dynamic changes in cell shape and positions with changes in subcellular localization of the fusion proteins. This will tie in with the (vertex-type) modeling of tissue mechanics, that are being developed in Hufnagel’s and in Shraiman’s labs, both of whom will provide theory supervision.
Project (4) Analyzing variation, robustness and compensation during embryogenesis. (Joel Rothman, UCSB). Variant initial conditions, molecular noise, and environmental conditions mean that every individual embryo in a complex animal follows a unique developmental pathway that nevertheless culminates in a similar final product. This profound variation seen between individuals has recently also been observed in simpler animals, such as C. elegans, that were renowned for their developmental constancy. Natural variation in C. elegans will be studied in developing embryos and individual cells and cellular substructures tagged with fluorescent proteins. Genetic alterations (e.g., mutants defective for buffering systems), laser-microsurgery, temperature gradients, and chemical perturbants, will be used to test the plasticity of individual cellular events (cell division timing, position, shape, and organelle distribution). Computational modeling will be performed to identify at least one step or process that drives deviations in cellular geometry toward a normalized state, resulting in reproducible output of embryogenesis.
Project (5) Mechanics of tissue morphogenesis in Drosophila (Otger Campas, UCSB). Morphogenesis involves tissue growth and remodeling in space and time. This project will introduce the students to the cutting-edge techniques developed for quantitatively measuring mechanics and collective cellular movements in living tissues. We will focus on early Drosophila embryogenesis to study cellular motions and cell shape changes during gastrulation. Students will characterize tissue flow quantitatively by analyzing correlations in cellular movements that define their collective behavior. Tissue flows will be perturbed using UV laser pulses to disrupt cellular junctions. Quantitative analysis of the tissue flow after laser ablations provides quantitative information about local tissue mechanics, which can be interpreted with the help of the vertex-type models. This project will provide the students the necessary quantitative methods to analyze tissue mechanics in other systems.
Project (6) Single cell studies of stem cell differentiation dynamics. (Pierre Neveu, EMBL). This project will study differentiation of mouse embryonic stem cell into a neural lineage. We will use ESC lines expressing fluorescent proteins reporting expression of miRNAs (excellent markers of cell states) and transcription factors associated with pluripotency and neurogenesis. To probe the differentiation landscape, we will use ESC lines where we can perturb the expression of key neurogenesis regulators. Students will perform automated quantitative single cell live imaging of neural differentiation under various conditions. Movies will be segmented and tracked to extract the behavior of single cells. The data will be used to differentiate between two quantitative models: one based on the assumption of exchange of stability between two attractor fixed points; the other based on bistability.