Application deadline February 16, 2025
Course Subject:
As animals move their bodies to explore the world, they must integrate sensory and motor information. Course participants will conduct experiments in different invertebrate organisms and sensory systems to map the neuronal circuits and algorithmic principles underlying the sensory-motor control of active sensing. Research projects will include the design of new behavioral assays, behavior quantification, manipulation and measurement of neural activity, and advanced data analysis to develop physical models of behavioral control.
Course Directors:
Instructors:
Guest Lecturers (partial list):
The summer course is closely linked to the concurrent KITP program Neurophysics of Active Sensing. Course participants will attend the program's daily research seminars as part of the course curriculum. Students and lecturers will also have frequent opportunities for less formal interactions. Confirmed program participants include Florian van Breugel (UNR), Lisa Fenk (MPI-BI), David Kleinfeld (UCSD), Leo Petreanu (Champalimaud), Kathy Nagel (NYU), Pavan Ramdya (EPFL), Dima Rinberg (NYU), Monika Scholz (MPI-CAESAR), Tanya Tabachnik (Zuckerman Inst.) and Massimo Vergassola (UCSD).
Course Structure
The structure for this five-week school follows the model established in the 2018 course on sensory navigation and 2022 course on locomotion. The course will be closely linked to the concurrent KITP program "Neurophysics of Active Sensing", with students attending program talks each morning, as well as joint social events. Interaction with program speakers will also be facilitated through tutorial sessions and informal meetings over coffee and meals. Afternoon are dedicated to lab work with ample time for data collection and analysis. Teams of 4 students will work with 2 instructors and a teaching assistant on a research project for the full 5 weeks of the school. Students will also have room to explore their own interests, based on initial instructor-guided projects. Students in different modules will interact informally as many research areas benefit from comparison or use similar technical approaches. The philosophy of the school fosters knowledge sharing between students and instructors. Students actively teach and learn from each other, drawing on their diverse areas of expertise. Project groups are intentionally composed of students from different academic backgrounds, encouraging cross-pollination of ideas. Additionally, students work closely with instructors, teaching assistants, and guest lecturers from the associated KITP program, leveraging their research experience. At the end of the course, the outcome of the research projects will be presented to the KITP program for feedback.
A typical day's schedule includes:
- 2 morning program talks at KITP
- Lunch with other course and program participants
- Afternoon lab work
- Dinner in a dining hall, followed by evening lab work or an informal lecture or science discussion.
On weekends, students can attend program BBQs and self-organize outings to the beach or around Santa Barbara.
Accommodations, Fees and Financial Assistance
Students are housed in double-occupancy rooms in UCSB student apartments and provided with 19-meal plan at campus dining commons. The room and board fee is $2861. There are no other fees or tuition. Students who need financial assistance can request it in their course application form; financial need does not affect an applicant's chances of admission. A limited number of single rooms are available for a $1462 supplement.
Experimental Projects
A neural substrate for efference copy
Co-Instructors: Brad Dickerson* and Eugenia Chiappe
*module leader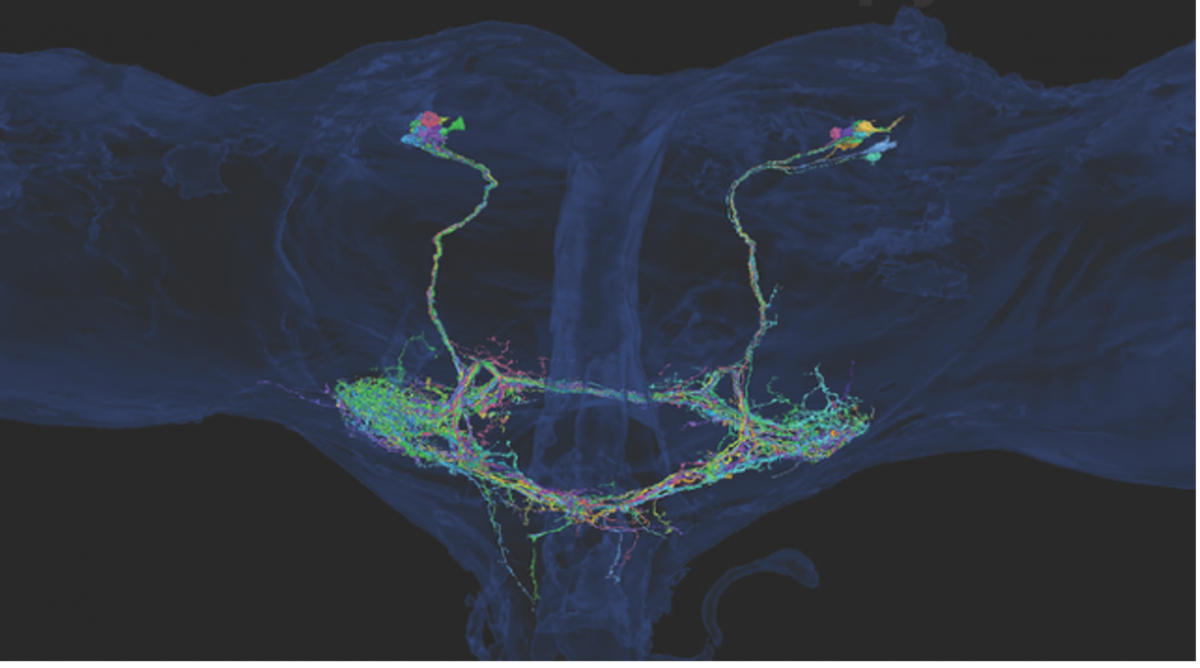
This module aims to uncover the neural mechanisms underlying the integration of self-generated motion and external sensory cues during voluntary sensorimotor behavior in flies. Previous work has shown that the fly visual system utilizes an "efference copy" signal to briefly attenuate the responses of motion-sensitive neurons during active flight maneuvers, but the origin of this signal remains unclear. We hypothesize that the fly's halteres, specialized structures that provide crucial feedback for flight control, transmit inhibitory signals to visual interneurons to enable the distinction between self-motion and external perturbations. By combining recent connectome data and genetic tools, we have identified a population of neurons, termed cLPTCrns, that provide this inhibitory input from the halteres to motion-sensitive neurons like the lobula plate tangential cells and ocelli. The goal of this module is to examine how manipulating the activity of these neurons affects the flies' ability to execute rapid, voluntary turning maneuvers (saccades) in the context of self-generated optic flow.
Understanding the functions of the descending information from mechanosensors in antennae to the haltere motor system
Co-Instructors: Jessica Fox*, Sung Soo Kim and Marie Suver
*module leader
This module aims to elucidate the neural mechanisms underlying the control of haltere motion in flies. Previous work has shown that descending interneurons (DNs) in the central brain can modulate haltere kinematics, thereby indirectly regulating the mechanosensory feedback that drives wing and head movements
during flight. The research plan involves two key objectives: 1) selectively activating or inactivating DN populations, particularly the DNp17 neurons that project exclusively to the haltere neuropil, while observing resulting changes in haltere, head, and wing movements using high-speed videography; and 2) directly measuring the responses of these DNs to antennal airflow stimulation during tethered flight, using two-photon calcium imaging. These experiments are designed to engage students in a wide range of neuroscience research techniques, including anatomy, behavior, physiology, optogenetics, and data analysis, ultimately mapping the neural circuits linking brain activity to the control of flight-related behaviors in insects.
Evolution of the neuromechanics of active sensing in the Drosophila larva
Co-Instructors: Ellie Heckscher*, Matthieu Louis, Akinao Nose, Nikolaos Papachatzis and Madhu Venkadesan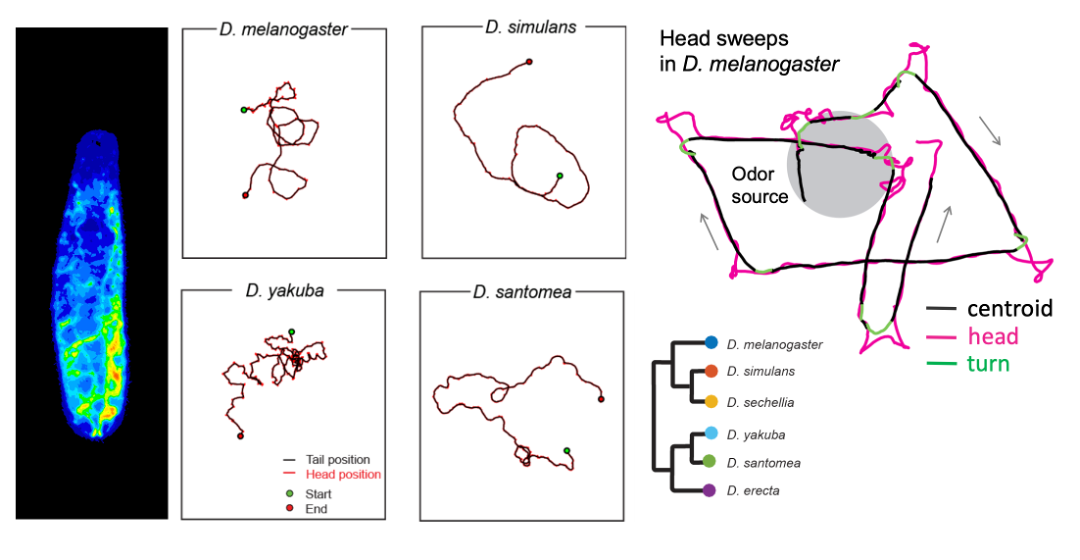
*module leader
For survival, larval fruit flies must successfully navigate through their environments—locating nutritive food sources and avoiding biotic (e.g., predators) and abiotic (e.g., heat) threats using active sensing behaviors. One of the best understood of these behaviors in Drosophila melanogaster larvae is a head sweep (or head cast) that moves sensory organs laterally to scan the environment. Active sensing mechanisms rely on the larva’s ability to accurately monitor its body position in space (i.e., proprioception). Yet, how active sensing interacts with organismal biophysics and self-sensing remains elusive. We will approach this question by comparing the active sensing behaviors across different species of Drosophila and other related insect species and through mathematical analyses of self-motion-induced flow and elastic deformation of the body that affects proprioception. We will consider orientation responses to two sensory modalities: chemotaxis and phototaxis. In particular, we will examine whether and how the evolution of sensory systems and proprioception have contributed to active sensing to adapt to the properties of distinct ecological niches.
Figure: Drosophila larva (left). Examples of larval trajectories of four Drosophila species on an agarose substrate with the same stiffness (Elie Fink, Louis lab). Illustration of head sweep in D. melanogaster (right) from Gomez-Marin et al., Nat. Com. 2011.
Navigating in the Extremes: Exploring Sensory-Driven Taxis and Cryptobiosis Recovery in Tardigrades
Co-instructors: Molly Kirk and Ana Lyons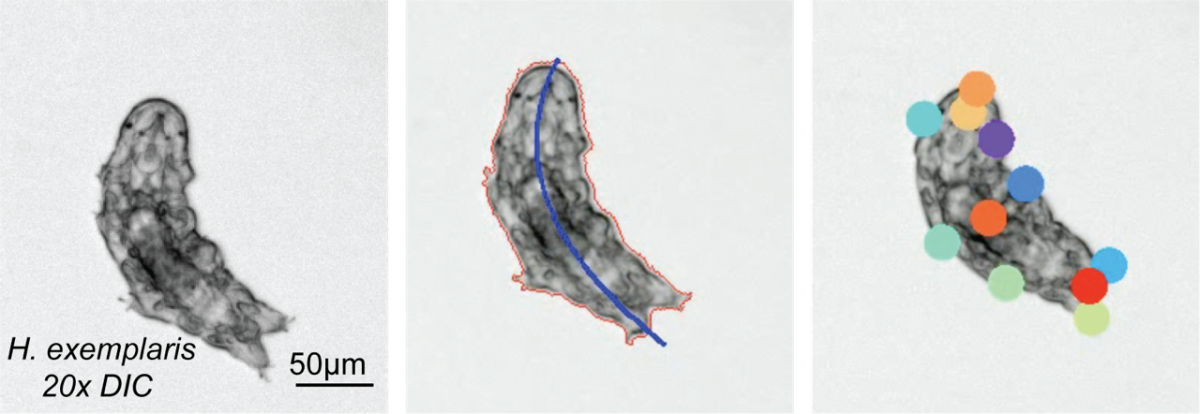
In this module, we will explore sensory-driven navigation (taxis) in tardigrade, Hypsibius exemplaris. Tardigrades are extraordinary micro-animals capable of withstanding extreme environmental conditions, including near-complete anoxia, desiccation, freezing in the absence of cryoprotectants, and even the vacuum and ionizing radiation of space. With a compact nervous system of approximately 200 neurons, tardigrades represent a simple yet powerful model to study sensory responses in environments that would be lethal to most other organisms. This module will investigate how tardigrades respond to various stimuli—optical, chemical, thermal, etc.—by identifying their behavioral strategies for taxis. Ultimately, we will explore how these navigational strategies change during recovery from cryptobiosis, a dormant state entered in response to extreme environmental stress, providing insights into the resilience of sensory systems in harsh conditions.
The module will focus on three unexplored areas of tardigrade neurobiology. (i) Valence Identification: By observing behaviors responding to various chemicals, light intensities, and temperature gradients, we will characterize the positive or negative associations that drive tardigrade movement. (ii) Behavioral Strategy: Through detailed behavioral tracking, students will learn to identify the distinct navigational strategies tardigrades employ when approaching or avoiding stimuli. (iii) Cryptobiosis Recovery Investigation: Lastly, the course will explore how sensory valences and navigational strategies are altered by cryptobiosis. Throughout the module, students will employ quantitative tracking techniques and analysis to understand how even simple nervous systems coordinate complex behaviors.
Antennal dynamics and active sensing in ants
Co-instructors: Venkatesh Murthy* and Orit Peleg
*module leader
Ants, like many other insects, use their antennae actively to explore the world. Since they are largely terrestrial insects, they sample signals close to the ground – these signals are often in the form of chemicals. Trail following is characteristic behavior in many ants, and in this module, we will examine how ants follow trails deliberately constructed in the lab to allow precise quantification. Using videography, computer vision and computational ethology, students in this module will characterize trail following phenomena, focusing on antennal dynamics. Questions can center on the biology of trail following, empirical computational analysis to describe the behavior, or theoretical formulations to uncover algorithms used by ants to follow trails. In addition to behavior of solitary ants, students can also explore trail following by groups of ants to study collective behavior.
What is the KITP QBio Summer Research Course?
Understanding fundamental problems in biology requires both advanced experiments and the quantitative/theoretical approach traditionally taken by physics. The Santa Barbara Advanced School of Quantitative Biology “QBio” summer course trains early-career scientists to integrate these different approaches by having them do it all–they take part in novel, team-taught lab experiments led by renowned faculty; they integrate quantitative modeling and theory into their experimental design and data analysis; and they become part of an interdisciplinary community of biologists and physicists. The course aims to teach students to communicate and collaborate with people with different and complementary backgrounds: eliminating the scientific language barrier is a major goal of the course. Students leave the course with an expanded network of peers, senior program participants and course instructors. Ideally, students emerge from the course with much broader scientific horizons and confidence in their own ability to chart the path of their own research.
Who should apply?
The course is geared toward advanced graduate students and postdocs, whose rigorous disciplinary training and significant research experience has prepared them to absorb material through high-level seminars and a research environment. The high instructor-to-student ratio and team-based project format create a supportive environment, but the course is intensive and fast-paced. With only 5 weeks to work, students must be self-driven to get results. Since participants do much of their learning from teammates and through informal interactions with program participants, openness to others’ ideas (particularly those from different scientific and cultural backgrounds) is crucial, and the confidence to initiate conversations with senior scientists help successful students make the most of their time at KITP. Successful applicants typically show curiosity, a clear idea of how the course will impact their research program, a desire to do interdisciplinary work, and strong quantitative skills.
Last day #kitpqbio. After of 5 weeks of 24/7 work, students present their incredible achievements. Pure science! Then bbq at the legendary Munger residence. What a summer! Thanks @KITP_UCSB! pic.twitter.com/e5yq25re0W— Xavier Trepat (@XavierTrepat) August 25, 2023
What do students say about the course?
"Participating in research projects outside my PhD work and interacting with other researchers revealed that - (a) I am broadly curious about biology, (b) I can ask questions and formulate testable hypothesis and (c) I enjoy science. When one of the instructors admired my efforts in his project and offered me a postdoc position, I realized that my abilities to do science were greater than my perception and decided to develop them further by continuing in research." - Sapna Chhabra (2019)
“I think one of the greatest values of this course has been the ability to interact with people from different fields and be able to learn the jargon that they use in one field and understand it at a deep enough level that we can then have conversations and build on that. Being able to interact with the physicists, theorists, and other experimentalists has helped broaden my knowledge base and also made me more comfortable, as a student, being able to talk across different fields and learn from people doing very different types of research, but all interested in and centered around different kinds of questions.” - Jess Kanwal (2018)
“For me, the project was very helpful for understanding the gap between the idealized data we theorize about and the data we actually have, and the project and tutorials left me much more versed in common computational techniques for bacterial genomics. The morning talks and the connections built over many hours with the other students in lab and over meals was also inspiring and makes me more likely to continue pursuing an academic career.” - Michael McLaren (2017)
“The KITP/QBio summer course is quite unique in the world and has been a true accelerator for my career. It first gave me a broad, comprehensive and timely overview on the field of morphogenesis, from both physics and biology points of view. This has been critical to nurture my own research project and to successfully apply to group leader positions. As a theoretician, the school allowed me to perform my first biological experiments ever. I realized that 1) I was actually not too bad in doing experiments, 2) I’d probably very much like doing my own ones one day. . . . The practicals in small groups, mixing biologists and physicists was a fantastic opportunity to learn from each others and to gain better teaching skills. This was a perfect mix of theory and experiments, and a perfect setting to make emerge original research ideas.” - Herve Turlier (2016)
Spent amazing five weeks @KITP_UCSB during #morpho23 and #kitpqbio. I enjoyed the experimental work with "TEAM HANGRY", instructed by @viktri08 and @KStapornwongkul. Can't wait to be back @BauschLab to try new ideas, I got during discussions within this stimulating environment. pic.twitter.com/EbrF2KYGvW
— Marion Raich (@marion_raich) August 28, 2023